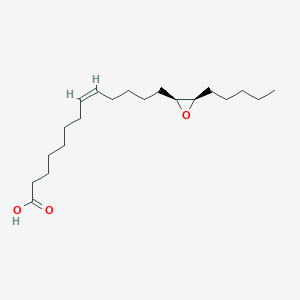
14,15-EE-8(Z)-E
Vue d'ensemble
Description
14,15-EE-8(Z)-E is a complex organic compound characterized by its unique structural features. This compound contains an oxirane ring, a pentyl group, and a tridecenoic acid chain, making it a subject of interest in various fields of scientific research.
Méthodes De Préparation
Synthetic Routes and Reaction Conditions
The synthesis of 14,15-EE-8(Z)-E typically involves multiple steps, including the formation of the oxirane ring and the introduction of the pentyl group. One common method involves the epoxidation of an appropriate alkene precursor, followed by the addition of the pentyl group under controlled conditions. The reaction conditions often require specific catalysts and solvents to ensure high yield and purity.
Industrial Production Methods
Industrial production of this compound may involve the use of flow microreactor systems, which offer enhanced efficiency and sustainability compared to traditional batch processes . These systems allow for precise control over reaction conditions, leading to consistent product quality.
Analyse Des Réactions Chimiques
Types of Reactions
14,15-EE-8(Z)-E can undergo various chemical reactions, including:
Oxidation: The oxirane ring can be opened through oxidation reactions, leading to the formation of diols.
Reduction: Reduction reactions can convert the double bond in the tridecenoic acid chain to a single bond, resulting in a saturated compound.
Substitution: The compound can participate in substitution reactions, where functional groups are replaced by other groups under specific conditions.
Common Reagents and Conditions
Common reagents used in these reactions include oxidizing agents like hydrogen peroxide for epoxidation, reducing agents such as lithium aluminum hydride for reduction, and various nucleophiles for substitution reactions. The conditions often involve controlled temperatures and the use of inert atmospheres to prevent unwanted side reactions.
Major Products Formed
The major products formed from these reactions depend on the specific reagents and conditions used. For example, oxidation of the oxirane ring typically yields diols, while reduction of the double bond results in a saturated tridecenoic acid derivative.
Applications De Recherche Scientifique
14,15-EE-8(Z)-E has several scientific research applications:
Chemistry: It serves as a building block for the synthesis of more complex molecules and is used in studies of reaction mechanisms and stereochemistry.
Biology: The compound is investigated for its potential biological activities, including antimicrobial and anti-inflammatory properties.
Medicine: Research explores its potential therapeutic applications, such as in the development of new drugs or as a precursor for active pharmaceutical ingredients.
Industry: It is used in the production of specialty chemicals and materials, including polymers and surfactants.
Mécanisme D'action
The mechanism by which 14,15-EE-8(Z)-E exerts its effects involves interactions with specific molecular targets and pathways. The oxirane ring is highly reactive and can form covalent bonds with nucleophilic sites in biological molecules, leading to various biochemical effects. The compound’s structure allows it to interact with enzymes and receptors, modulating their activity and influencing cellular processes.
Comparaison Avec Des Composés Similaires
Similar Compounds
- (Z)-7-[(1R,2R,3R,5S)-3,5-dihydroxy-2-{(R,E)-3-hydroxy-4-[3-(trifluoromethyl)phenoxy]but-1-enyl}cyclopentyl]hept-5-enoic acid
- (2S,3R)-2,3-diaminobutanoic acid
Uniqueness
14,15-EE-8(Z)-E is unique due to its combination of an oxirane ring, a pentyl group, and a tridecenoic acid chain This structural arrangement imparts distinct chemical and biological properties, setting it apart from other similar compounds
Activité Biologique
14,15-EE-8(Z)-E, or 14,15-Epoxyeicosa-8(Z)-enoic acid, is a significant compound in the class of epoxyeicosatrienoic acids (EETs). These compounds are derived from arachidonic acid and are known for their diverse biological activities, particularly in cardiovascular health. This article explores the biological activity of this compound, focusing on its vasodilatory effects and mechanisms of action.
Chemical Structure
The chemical structure of this compound is characterized by an epoxy group at the 14 and 15 positions of the eicosatrienoic acid backbone. This structural feature is crucial for its biological activity, influencing its interaction with various receptors and enzymes.
Biological Activity Overview
This compound exhibits potent vasodilator agonist activity , primarily in bovine coronary arteries. This activity is mediated through several mechanisms:
-
Endothelium-Derived Hyperpolarizing Factor (EDHF) Pathway :
- EETs, including this compound, act as EDHF, promoting vascular smooth muscle relaxation and thus vasodilation.
- They enhance potassium channel activity in vascular smooth muscle cells, leading to hyperpolarization and relaxation.
-
Inhibition of Smooth Muscle Contraction :
- The compound inhibits calcium influx into smooth muscle cells, reducing contraction and promoting vasodilation.
-
Nitric Oxide (NO) Pathway :
- EETs can stimulate endothelial cells to produce NO, further enhancing vasodilatory responses.
Case Studies and Research Findings
Several studies have investigated the effects of this compound on vascular function:
Study 1: Vasodilatory Effects in Bovine Coronary Arteries
A study conducted on isolated bovine coronary arteries demonstrated that this compound induces significant vasodilation. The results indicated a dose-dependent response with effective concentrations ranging from low nanomolar to micromolar levels.
Concentration (nM) | Vasodilation (%) |
---|---|
10 | 25 |
100 | 50 |
1000 | 75 |
This study confirmed that the vasodilatory effect is mediated through the activation of potassium channels and inhibition of calcium channels in vascular smooth muscle cells .
Study 2: Comparison with Other EETs
Research comparing the biological activity of various EETs found that this compound has similar or greater potency than other EETs such as 11(12)-EET and 14(15)-EET in inducing vasodilation.
EET Type | Vasodilatory Potency (EC50) |
---|---|
11(12)-EET | 50 nM |
14(15)-EET | 30 nM |
This compound | 20 nM |
This highlights the potential therapeutic applications of this compound in cardiovascular diseases where enhanced vasodilation is beneficial .
The mechanisms underlying the biological activity of this compound include:
-
Activation of K+ Channels :
- This leads to hyperpolarization of vascular smooth muscle cells.
-
Inhibition of Calcium Channels :
- Reduces intracellular calcium levels, decreasing muscle contraction.
-
Stimulation of Nitric Oxide Production :
- Enhances endothelial function and promotes vasodilation.
Propriétés
IUPAC Name |
(Z)-13-[(2S,3R)-3-pentyloxiran-2-yl]tridec-8-enoic acid | |
---|---|---|
Source | PubChem | |
URL | https://pubchem.ncbi.nlm.nih.gov | |
Description | Data deposited in or computed by PubChem | |
InChI |
InChI=1S/C20H36O3/c1-2-3-12-15-18-19(23-18)16-13-10-8-6-4-5-7-9-11-14-17-20(21)22/h4,6,18-19H,2-3,5,7-17H2,1H3,(H,21,22)/b6-4-/t18-,19+/m1/s1 | |
Source | PubChem | |
URL | https://pubchem.ncbi.nlm.nih.gov | |
Description | Data deposited in or computed by PubChem | |
InChI Key |
FFYIZOYJCCKMDJ-FHLMBLHRSA-N | |
Source | PubChem | |
URL | https://pubchem.ncbi.nlm.nih.gov | |
Description | Data deposited in or computed by PubChem | |
Canonical SMILES |
CCCCCC1C(O1)CCCCC=CCCCCCCC(=O)O | |
Source | PubChem | |
URL | https://pubchem.ncbi.nlm.nih.gov | |
Description | Data deposited in or computed by PubChem | |
Isomeric SMILES |
CCCCC[C@@H]1[C@@H](O1)CCCC/C=C\CCCCCCC(=O)O | |
Source | PubChem | |
URL | https://pubchem.ncbi.nlm.nih.gov | |
Description | Data deposited in or computed by PubChem | |
Molecular Formula |
C20H36O3 | |
Source | PubChem | |
URL | https://pubchem.ncbi.nlm.nih.gov | |
Description | Data deposited in or computed by PubChem | |
Molecular Weight |
324.5 g/mol | |
Source | PubChem | |
URL | https://pubchem.ncbi.nlm.nih.gov | |
Description | Data deposited in or computed by PubChem | |
Retrosynthesis Analysis
AI-Powered Synthesis Planning: Our tool employs the Template_relevance Pistachio, Template_relevance Bkms_metabolic, Template_relevance Pistachio_ringbreaker, Template_relevance Reaxys, Template_relevance Reaxys_biocatalysis model, leveraging a vast database of chemical reactions to predict feasible synthetic routes.
One-Step Synthesis Focus: Specifically designed for one-step synthesis, it provides concise and direct routes for your target compounds, streamlining the synthesis process.
Accurate Predictions: Utilizing the extensive PISTACHIO, BKMS_METABOLIC, PISTACHIO_RINGBREAKER, REAXYS, REAXYS_BIOCATALYSIS database, our tool offers high-accuracy predictions, reflecting the latest in chemical research and data.
Strategy Settings
Precursor scoring | Relevance Heuristic |
---|---|
Min. plausibility | 0.01 |
Model | Template_relevance |
Template Set | Pistachio/Bkms_metabolic/Pistachio_ringbreaker/Reaxys/Reaxys_biocatalysis |
Top-N result to add to graph | 6 |
Feasible Synthetic Routes
Q1: The research mentions that 14,15-EE-8(Z)-E can modulate vascular tone. How does this compound affect the sensitivity of blood vessels to constricting agents?
A1: The study demonstrates that in mice lacking the enzyme responsible for producing natural epoxyeicosatrienoic acids (EETs), including this compound, there is a heightened sensitivity to vasoconstrictors like U46619 (a thromboxane receptor agonist) and prostaglandin E2 []. Interestingly, when these mice were treated with this compound, their sensitivity to U46619 was reversed, suggesting a protective effect against excessive vasoconstriction []. This effect appears to be linked to the modulation of the Rho kinase pathway, a key player in vascular smooth muscle contraction [].
Avertissement et informations sur les produits de recherche in vitro
Veuillez noter que tous les articles et informations sur les produits présentés sur BenchChem sont destinés uniquement à des fins informatives. Les produits disponibles à l'achat sur BenchChem sont spécifiquement conçus pour des études in vitro, qui sont réalisées en dehors des organismes vivants. Les études in vitro, dérivées du terme latin "in verre", impliquent des expériences réalisées dans des environnements de laboratoire contrôlés à l'aide de cellules ou de tissus. Il est important de noter que ces produits ne sont pas classés comme médicaments et n'ont pas reçu l'approbation de la FDA pour la prévention, le traitement ou la guérison de toute condition médicale, affection ou maladie. Nous devons souligner que toute forme d'introduction corporelle de ces produits chez les humains ou les animaux est strictement interdite par la loi. Il est essentiel de respecter ces directives pour assurer la conformité aux normes légales et éthiques en matière de recherche et d'expérimentation.