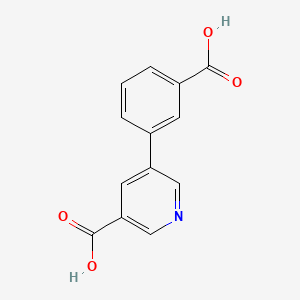
5-(3-Carboxyphenyl)nicotinic acid
Vue d'ensemble
Description
5-(3-Carboxyphenyl)nicotinic acid (CAS: 1261976-89-9, molecular formula: C₁₃H₉NO₄) is a nicotinic acid derivative featuring a phenyl ring substituted with a carboxylic acid group at the 3-position and a pyridine ring with a carboxylic acid group at the 3-position (nicotinic acid scaffold) . It is primarily utilized in coordination chemistry as a building block for metal-organic frameworks (MOFs) and coordination polymers due to its bifunctional carboxylate groups, which facilitate diverse metal-ligand interactions . Current safety data indicate moderate acute oral toxicity (H302), skin irritation (H315), and respiratory irritation (H335), necessitating proper personal protective equipment during handling .
Méthodes De Préparation
Oxidation of Methyl-Substituted Precursors
Hydrogen Peroxide/Sulfuric Acid-Mediated Oxidation
A widely adopted method involves oxidizing methyl-substituted pyridine derivatives to their corresponding carboxylic acids. In the context of 5-(3-Carboxyphenyl)nicotinic acid, this approach could employ 5-(3-carboxyphenyl)-3-methylpyridine as a precursor. The oxidation is conducted using a hydrogen peroxide (H₂O₂) and concentrated sulfuric acid (H₂SO₄) system, which selectively converts methyl groups to carboxylic acids without over-oxidizing adjacent functionalities .
Reaction Conditions:
-
Solvent: Concentrated H₂SO₄ (98%)
-
Oxidant: 30% H₂O₂
-
Temperature: 110–130°C
-
Time: 15–20 hours
This method benefits from mild conditions and avoids the use of toxic oxidants like potassium permanganate. The limited oxidation capability of H₂O₂ prevents simultaneous oxidation of multiple methyl groups, enhancing selectivity . Post-reaction workup involves pH adjustment to 3–4, precipitating the product for isolation.
The Suzuki-Miyaura reaction enables the introduction of a 3-carboxyphenyl group to the nicotinic acid scaffold. This method requires a halogenated nicotinic acid derivative (e.g., 5-bromonicotinic acid) and 3-carboxyphenylboronic acid.
Typical Protocol:
-
Catalyst: Pd(PPh₃)₄ (5 mol%)
-
Base: Na₂CO₃
-
Solvent: Dimethylformamide (DMF)/water (4:1)
-
Temperature: 80–100°C
-
Time: 12–24 hours
The reaction proceeds via a palladium-mediated transmetallation mechanism, forming a carbon-carbon bond between the aryl boronic acid and the nicotinic acid halide. Post-coupling hydrolysis of protecting groups (if present) yields the target compound.
Ullmann-Type Coupling
Copper-catalyzed Ullmann couplings offer an alternative for attaching the 3-carboxyphenyl group. This method is suitable for substrates with poor reactivity in Suzuki reactions.
Representative Conditions:
-
Catalyst: CuI (10 mol%)
-
Ligand: 1,10-Phenanthroline
-
Base: K₃PO₄
-
Solvent: DMSO
-
Temperature: 120°C
While less efficient than palladium-based methods, Ullmann couplings avoid sensitive boronic acid precursors.
Carboxylation of Pre-Organized Intermediates
Kolbe-Schmitt Carboxylation
The Kolbe-Schmitt reaction introduces carboxyl groups onto aromatic rings via electrophilic substitution. For 5-phenylnicotinic acid derivatives, this method could carboxylate the phenyl ring at the meta position.
Key Parameters:
This route is limited by regioselectivity challenges and requires precise control of reaction conditions to favor meta-substitution.
Comparative Analysis of Synthetic Routes
Emerging Hydrothermal Synthesis Approaches
Recent advances in coordination polymer synthesis have demonstrated the utility of hydrothermal conditions for stabilizing reactive intermediates. A 3D iron-based coordination polymer synthesized from 5-(4’-carboxyphenoxy)nicotinic acid highlights the potential for adapting similar strategies to this compound .
Hydrothermal Protocol:
Analyse Des Réactions Chimiques
Types of Reactions
5-(3-Carboxyphenyl)nicotinic acid undergoes various chemical reactions, including:
Oxidation: The carboxylic acid groups can be oxidized to form corresponding carboxylates.
Reduction: The nitro group, if present, can be reduced to an amine.
Substitution: The aromatic ring can undergo electrophilic substitution reactions.
Common Reagents and Conditions
Oxidation: Common oxidizing agents include potassium permanganate and chromium trioxide.
Reduction: Reducing agents such as sodium borohydride and hydrogen gas in the presence of a catalyst are used.
Substitution: Electrophilic substitution reactions often use reagents like bromine or chlorine in the presence of a Lewis acid catalyst.
Major Products Formed
The major products formed from these reactions depend on the specific conditions and reagents used. For example, oxidation typically yields carboxylates, while reduction can produce amines.
Applications De Recherche Scientifique
Medicinal Chemistry
1. Anti-inflammatory Properties
Recent studies have indicated that nicotinic acid derivatives, including 5-(3-Carboxyphenyl)nicotinic acid, exhibit significant anti-inflammatory effects. Research has shown that niacin can reduce inflammatory markers such as C-reactive protein (CRP) and tumor necrosis factor-alpha (TNF-α) in patients with conditions like type 2 diabetes and cardiovascular diseases. A systematic review highlighted that high-dose niacin administration led to notable reductions in these inflammatory markers, suggesting potential therapeutic roles for its derivatives in managing inflammation-related diseases .
2. Lipid Modulation
Nicotinic acid is widely recognized for its lipid-modifying effects. It is used to treat hyperlipidemia by reducing low-density lipoprotein cholesterol (LDL-C) and triglycerides while increasing high-density lipoprotein cholesterol (HDL-C). The mechanism involves the activation of G protein-coupled receptors that inhibit the release of free fatty acids from adipose tissue, thus lowering lipid synthesis in the liver . The derivative this compound may enhance these effects due to its structural modifications, potentially leading to improved therapeutic outcomes.
3. Antioxidant Activity
The antioxidant properties of nicotinic acid derivatives are also noteworthy. Studies have shown that these compounds can scavenge free radicals and reduce oxidative stress, which is implicated in various chronic diseases. This property makes this compound a candidate for further research in developing antioxidant therapies .
Agricultural Applications
1. Plant Growth Regulation
Research indicates that nicotinic acid derivatives can influence plant growth and development. They may act as growth regulators, enhancing seed germination and root development. Preliminary studies suggest that this compound could be effective in promoting plant health and resilience against environmental stresses .
2. Soil Health Improvement
Nicotinic acid derivatives have been explored for their potential to improve soil health by enhancing microbial activity. They may serve as organic amendments that promote beneficial soil microorganisms, leading to improved nutrient availability for plants. This application is particularly relevant in sustainable agriculture practices aimed at reducing chemical fertilizer use .
Materials Science Applications
1. Corrosion Inhibition
This compound has been investigated for its potential as a corrosion inhibitor in metal surfaces. Its ability to form protective films on metals can significantly enhance their resistance to corrosion, which is crucial for extending the lifespan of industrial equipment and infrastructure .
2. Polymer Chemistry
In polymer science, nicotinic acid derivatives are being studied for their role as additives that can modify the properties of polymers. They may improve thermal stability and mechanical strength, making them suitable for various industrial applications .
Case Study 1: Anti-inflammatory Effects of Niacin Derivatives
A randomized controlled trial involving patients with type 2 diabetes demonstrated that high-dose niacin significantly reduced CRP levels after 24 weeks of treatment. This study supports the potential use of niacin derivatives like this compound in clinical settings targeting inflammation .
Case Study 2: Soil Health Enhancement
A field experiment evaluated the effects of applying nicotinic acid-based amendments on soil microbial communities. Results showed a marked increase in beneficial bacteria populations and improved soil nutrient profiles, indicating the positive impact of such compounds on agricultural productivity .
Mécanisme D'action
The mechanism of action of 5-(3-Carboxyphenyl)nicotinic acid involves its interaction with specific molecular targets and pathways. For instance, nicotinic acid derivatives inhibit hepatocyte diacylglycerol acyltransferase-2, preventing the final step of triglyceride synthesis in hepatocytes . This leads to decreased production of low-density lipoproteins and very low-density lipoproteins.
Comparaison Avec Des Composés Similaires
Comparison with Structurally Similar Compounds
Structural Analogs and Substituent Effects
Key structural analogs include:
Key Observations :
- Substituent Position : The 3-carboxyphenyl group in the target compound enhances coordination versatility compared to 4-substituted analogs (e.g., 4-bromo or 4-acetyl), as the meta position allows for more flexible binding geometries in MOFs .
- Functional Groups: Halogenated analogs (e.g., 5-(4-Bromophenyl)) may improve thermal stability in MOFs but introduce higher toxicity risks .
Activité Biologique
5-(3-Carboxyphenyl)nicotinic acid is a derivative of nicotinic acid, known for its various biological activities. This compound has garnered attention due to its potential therapeutic applications, particularly in the fields of anti-inflammatory and anticancer treatments. This article reviews the biological activity of this compound, synthesizing findings from diverse studies and presenting relevant data.
Chemical Structure and Properties
This compound features a carboxyphenyl group attached to the 5-position of a nicotinic acid moiety. Its molecular formula is C12H11NO3, with a molecular weight of approximately 219.22 g/mol. The unique structural arrangement contributes to its distinct chemical properties, influencing its interaction with biological targets.
Mechanisms of Biological Activity
Research indicates that this compound exhibits significant biological activity through various mechanisms:
- Enzyme Inhibition : The compound has been shown to inhibit specific enzymes involved in metabolic pathways. For instance, it may interact with nicotinic acetylcholine receptors, influencing neurotransmitter release and cellular signaling processes .
- Anti-inflammatory Effects : Studies suggest that this compound can modulate inflammatory responses by inhibiting pro-inflammatory cytokines and promoting anti-inflammatory pathways .
- Antimicrobial Activity : Preliminary studies indicate potential antimicrobial properties against various bacterial strains, including Staphylococcus aureus. The Minimum Inhibitory Concentration (MIC) values for related compounds suggest that modifications in the structure can enhance antibacterial efficacy .
Table 1: Summary of Biological Activities
Case Study: Anti-inflammatory Properties
A study conducted by researchers focused on the anti-inflammatory effects of this compound. The results demonstrated that the compound significantly reduced levels of tumor necrosis factor-alpha (TNF-α) in vitro. This suggests its potential utility in treating inflammatory diseases such as rheumatoid arthritis or inflammatory bowel disease .
Case Study: Antimicrobial Efficacy
Another investigation assessed the antimicrobial activity of related compounds derived from nicotinic acid. The study found that derivatives with similar structures to this compound exhibited promising antibacterial properties against methicillin-resistant Staphylococcus aureus (MRSA), with MIC values indicating effective inhibition at low concentrations .
Q & A
Basic Research Questions
Q. What are the recommended safety protocols for handling 5-(3-Carboxyphenyl)nicotinic acid in laboratory settings?
- Methodological Answer : Use NIOSH/CEN-compliant respiratory protection (e.g., P95/P1 filters for low exposure, OV/AG/P99 for high exposure) and full-body PPE to avoid skin/eye contact. Ensure ventilation to prevent dust/aerosol formation. Store below 28°C in dry conditions and avoid drainage contamination. First-aid measures include immediate decontamination and medical consultation .
Q. What is the acute toxicity profile of this compound?
- Methodological Answer : Classified as H302 (harmful if swallowed), H315 (skin irritation), H319 (serious eye irritation), and H335 (respiratory irritation). Acute oral toxicity falls under OSHA GHS Category 3. No carcinogenicity is reported by IARC, ACGIH, or NTP. Toxicity studies should follow OECD 423 (acute oral) and 404 (skin irritation) guidelines .
Q. How should researchers address gaps in physicochemical data for this compound?
- Methodological Answer : Prioritize experimental determination of log Pow, solubility, and thermal stability using techniques like HPLC (for log Pow), differential scanning calorimetry (melting point), and UV-Vis spectroscopy (aqueous solubility). Reference analogs like nicotinic acid (mp 236°C) for preliminary assumptions .
Advanced Research Questions
Q. How can this compound be optimized as a ligand for metal-organic frameworks (MOFs)?
- Methodological Answer : Design MOFs by coordinating carboxylate and pyridyl groups with metals (e.g., In, Cu). For example, defect-engineered Cu-MOFs synthesized via solvothermal methods (DMF, 120°C) show enhanced CO2 adsorption. Structural validation requires single-crystal XRD and BET surface area analysis .
Q. What analytical strategies validate the purity and stability of synthesized derivatives?
- Methodological Answer : Combine LC-MS (for molecular weight confirmation), <sup>1</sup>H/<sup>13</sup>C NMR (structural integrity), and accelerated stability studies (40°C/75% RH for 6 months). Monitor degradation via HPLC with a C18 column and 0.1% formic acid mobile phase .
Q. How do reaction conditions influence the compound’s reactivity in esterification or metal coordination?
- Methodological Answer : Esterification (e.g., methyl ester synthesis) requires H2SO4 catalysis in methanol under reflux. For metal complexes (Ni, Co, Cd), optimize pH (6–8) and temperature (60–80°C) to favor monodentate vs. bridging coordination modes, confirmed via IR (COO<sup>−</sup> stretching at 1600–1650 cm<sup>−1</sup>) and XANES .
Q. What in vitro models are suitable for assessing its biological activity?
- Methodological Answer : Use HEK-293 or A549 cell lines for cytotoxicity (MTT assay, IC50 determination). For anti-inflammatory activity, measure TNF-α suppression in LPS-stimulated macrophages. Molecular docking (AutoDock Vina) predicts binding affinity to COX-2 or NAD<sup>+</sup> salvage pathway enzymes .
Q. Experimental Design & Data Contradictions
Q. How to resolve discrepancies in reported stability under acidic/basic conditions?
- Methodological Answer : Conduct pH-dependent stability studies (pH 2–12, 25°C) with UV-Vis monitoring (λmax 270 nm). For conflicting decomposition data (e.g., CO release vs. no data), use TGA-FTIR to identify gaseous byproducts .
Q. Why do MOFs synthesized with this ligand show variable gas adsorption capacities?
- Methodological Answer : Variability arises from defect density (quantified via positron annihilation spectroscopy) and ligand orientation (DFT calculations). For example, TJT-100’s CO2 uptake (3.2 mmol/g at 1 bar) depends on activation temperature (150–200°C) .
Q. How to mitigate batch-to-batch variability in large-scale synthesis?
- Methodological Answer : Implement continuous flow reactors with inline PAT (e.g., Raman spectroscopy) for real-time monitoring. Optimize crystallization using antisolvent addition (ethanol/water) and control nucleation via cooling rate (1–5°C/min) .
Q. Tables for Key Data
Propriétés
IUPAC Name |
5-(3-carboxyphenyl)pyridine-3-carboxylic acid | |
---|---|---|
Source | PubChem | |
URL | https://pubchem.ncbi.nlm.nih.gov | |
Description | Data deposited in or computed by PubChem | |
InChI |
InChI=1S/C13H9NO4/c15-12(16)9-3-1-2-8(4-9)10-5-11(13(17)18)7-14-6-10/h1-7H,(H,15,16)(H,17,18) | |
Source | PubChem | |
URL | https://pubchem.ncbi.nlm.nih.gov | |
Description | Data deposited in or computed by PubChem | |
InChI Key |
NQVZYGPMEZNLCN-UHFFFAOYSA-N | |
Source | PubChem | |
URL | https://pubchem.ncbi.nlm.nih.gov | |
Description | Data deposited in or computed by PubChem | |
Canonical SMILES |
C1=CC(=CC(=C1)C(=O)O)C2=CC(=CN=C2)C(=O)O | |
Source | PubChem | |
URL | https://pubchem.ncbi.nlm.nih.gov | |
Description | Data deposited in or computed by PubChem | |
Molecular Formula |
C13H9NO4 | |
Source | PubChem | |
URL | https://pubchem.ncbi.nlm.nih.gov | |
Description | Data deposited in or computed by PubChem | |
DSSTOX Substance ID |
DTXSID80687439 | |
Record name | 5-(3-Carboxyphenyl)pyridine-3-carboxylic acid | |
Source | EPA DSSTox | |
URL | https://comptox.epa.gov/dashboard/DTXSID80687439 | |
Description | DSSTox provides a high quality public chemistry resource for supporting improved predictive toxicology. | |
Molecular Weight |
243.21 g/mol | |
Source | PubChem | |
URL | https://pubchem.ncbi.nlm.nih.gov | |
Description | Data deposited in or computed by PubChem | |
CAS No. |
1261976-89-9 | |
Record name | 5-(3-Carboxyphenyl)pyridine-3-carboxylic acid | |
Source | EPA DSSTox | |
URL | https://comptox.epa.gov/dashboard/DTXSID80687439 | |
Description | DSSTox provides a high quality public chemistry resource for supporting improved predictive toxicology. | |
Avertissement et informations sur les produits de recherche in vitro
Veuillez noter que tous les articles et informations sur les produits présentés sur BenchChem sont destinés uniquement à des fins informatives. Les produits disponibles à l'achat sur BenchChem sont spécifiquement conçus pour des études in vitro, qui sont réalisées en dehors des organismes vivants. Les études in vitro, dérivées du terme latin "in verre", impliquent des expériences réalisées dans des environnements de laboratoire contrôlés à l'aide de cellules ou de tissus. Il est important de noter que ces produits ne sont pas classés comme médicaments et n'ont pas reçu l'approbation de la FDA pour la prévention, le traitement ou la guérison de toute condition médicale, affection ou maladie. Nous devons souligner que toute forme d'introduction corporelle de ces produits chez les humains ou les animaux est strictement interdite par la loi. Il est essentiel de respecter ces directives pour assurer la conformité aux normes légales et éthiques en matière de recherche et d'expérimentation.