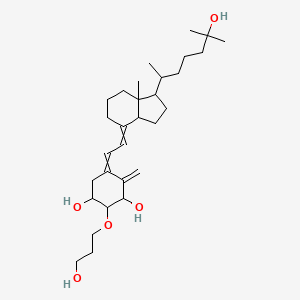
trans-Eldecalcitol
- Cliquez sur DEMANDE RAPIDE pour recevoir un devis de notre équipe d'experts.
- Avec des produits de qualité à un prix COMPÉTITIF, vous pouvez vous concentrer davantage sur votre recherche.
Vue d'ensemble
Description
Trans-Eldecalcitol, also known as this compound, is a useful research compound. Its molecular formula is C30H50O5 and its molecular weight is 490.725. The purity is usually 95%.
BenchChem offers high-quality this compound suitable for many research applications. Different packaging options are available to accommodate customers' requirements. Please inquire for more information about this compound including the price, delivery time, and more detailed information at info@benchchem.com.
Mécanisme D'action
Target of Action
Eldecalcitol, also known as trans-Eldecalcitol or 5-[2-[1-(6-Hydroxy-6-methylheptan-2-yl)-7a-methyl-2,3,3a,5,6,7-hexahydro-1H-inden-4-ylidene]ethylidene]-2-(3-hydroxypropoxy)-4-methylidenecyclohexane-1,3-diol, primarily targets the Vitamin D3 receptor (VDR) . The VDR plays a crucial role in calcium and phosphate metabolism, which are vital for bone health .
Mode of Action
Eldecalcitol is an analog of 1α,25-dihydroxyvitamin D3 [1,25 (OH)2D3], bearing a hydroxypropoxy residue at the 2β position . It binds to the VDR with less affinity but binds to the vitamin D-binding protein with higher affinity than 1,25 (OH)2D3, showing a long half-life in plasma . This interaction with its targets leads to changes in gene expression that influence calcium and phosphate metabolism .
Biochemical Pathways
Eldecalcitol affects several biochemical pathways. It has been found to suppress the PI3K/AKT/FOXOs pathway, which is involved in muscle atrophy . Additionally, it has been shown to inhibit the NF-κB signaling pathway, which plays a role in inflammation and immunity .
Result of Action
Eldecalcitol has been found to be effective in increasing bone mass and enhancing bone strength in rodents . It also effectively and safely increased lumbar and hip bone mineral density (BMD) in osteoporotic patients who also received vitamin D3 supplementation . Moreover, it has been associated with a higher risk of hypercalciuria .
Action Environment
Environmental factors can influence the action, efficacy, and stability of Eldecalcitol. For instance, food intake can affect the absorption of the drug . .
Analyse Biochimique
Biochemical Properties
Eldecalcitol plays a significant role in biochemical reactions. It has strong effects to reduce calcium reabsorption into the body from bones, therefore increasing bone mineral density . In animals, Eldecalcitol inhibits the activity of osteoclasts for the function to reduce bone degradation for calcium, while still able to maintain osteoblast function so as to not hinder bone formation .
Cellular Effects
Eldecalcitol influences cell function by inhibiting bone resorption and increasing bone mineral density (BMD) in osteoporotic patients . It also displays anti-tumor effect and inhibits cell proliferation, migration and induces apoptosis by suppressing GPx-1 .
Molecular Mechanism
Eldecalcitol exerts its effects at the molecular level through binding interactions with biomolecules. It inhibits the activity of osteoclasts, reducing bone degradation for calcium, while maintaining osteoblast function to not hinder bone formation .
Temporal Effects in Laboratory Settings
Over time, Eldecalcitol has been observed to increase bone mineral density and strengthen bone structure . It decreases bone reabsorption as observed through a bone reabsorption marker .
Dosage Effects in Animal Models
In animal models, the effects of Eldecalcitol vary with different dosages. It has been found to be a more potent inhibitor of bone resorption than alfacalcidol in an estrogen-deficient rat model of osteoporosis .
Metabolic Pathways
Eldecalcitol is involved in the metabolic pathway of vitamin D. It inhibits bone resorption and increases bone mineral density, effectively altering the metabolic flux of calcium in the body .
Propriétés
IUPAC Name |
5-[2-[1-(6-hydroxy-6-methylheptan-2-yl)-7a-methyl-2,3,3a,5,6,7-hexahydro-1H-inden-4-ylidene]ethylidene]-2-(3-hydroxypropoxy)-4-methylidenecyclohexane-1,3-diol |
Source
|
---|---|---|
Source | PubChem | |
URL | https://pubchem.ncbi.nlm.nih.gov | |
Description | Data deposited in or computed by PubChem | |
InChI |
InChI=1S/C30H50O5/c1-20(9-6-15-29(3,4)34)24-13-14-25-22(10-7-16-30(24,25)5)11-12-23-19-26(32)28(27(33)21(23)2)35-18-8-17-31/h11-12,20,24-28,31-34H,2,6-10,13-19H2,1,3-5H3 |
Source
|
Source | PubChem | |
URL | https://pubchem.ncbi.nlm.nih.gov | |
Description | Data deposited in or computed by PubChem | |
InChI Key |
FZEXGDDBXLBRTD-UHFFFAOYSA-N |
Source
|
Source | PubChem | |
URL | https://pubchem.ncbi.nlm.nih.gov | |
Description | Data deposited in or computed by PubChem | |
Canonical SMILES |
CC(CCCC(C)(C)O)C1CCC2C1(CCCC2=CC=C3CC(C(C(C3=C)O)OCCCO)O)C |
Source
|
Source | PubChem | |
URL | https://pubchem.ncbi.nlm.nih.gov | |
Description | Data deposited in or computed by PubChem | |
Molecular Formula |
C30H50O5 |
Source
|
Source | PubChem | |
URL | https://pubchem.ncbi.nlm.nih.gov | |
Description | Data deposited in or computed by PubChem | |
Molecular Weight |
490.7 g/mol |
Source
|
Source | PubChem | |
URL | https://pubchem.ncbi.nlm.nih.gov | |
Description | Data deposited in or computed by PubChem | |
Avertissement et informations sur les produits de recherche in vitro
Veuillez noter que tous les articles et informations sur les produits présentés sur BenchChem sont destinés uniquement à des fins informatives. Les produits disponibles à l'achat sur BenchChem sont spécifiquement conçus pour des études in vitro, qui sont réalisées en dehors des organismes vivants. Les études in vitro, dérivées du terme latin "in verre", impliquent des expériences réalisées dans des environnements de laboratoire contrôlés à l'aide de cellules ou de tissus. Il est important de noter que ces produits ne sont pas classés comme médicaments et n'ont pas reçu l'approbation de la FDA pour la prévention, le traitement ou la guérison de toute condition médicale, affection ou maladie. Nous devons souligner que toute forme d'introduction corporelle de ces produits chez les humains ou les animaux est strictement interdite par la loi. Il est essentiel de respecter ces directives pour assurer la conformité aux normes légales et éthiques en matière de recherche et d'expérimentation.