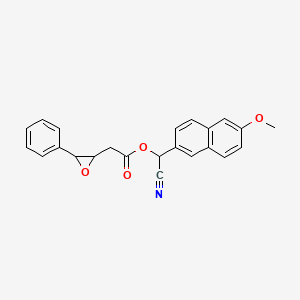
Phome
- Cliquez sur DEMANDE RAPIDE pour recevoir un devis de notre équipe d'experts.
- Avec des produits de qualité à un prix COMPÉTITIF, vous pouvez vous concentrer davantage sur votre recherche.
Vue d'ensemble
Description
Phome is a type of small molecule that is used to study the biochemical and physiological effects of a variety of substances. It is a synthetic molecule that has been developed for use in laboratory experiments and is used to study the effects of various molecules on the body. This compound is an important tool in the study of the effects of drugs, toxins, and other molecules on the body. It is also used to study the biochemical and physiological effects of various substances on the body.
Applications De Recherche Scientifique
Metabolic Engineering of Poly(3-Hydroxyalkanoates) From DNA to Plastic
:
- Summary: This paper discusses the potential applications of poly(3-hydroxyalkanoates) (PHAs), a class of microbially produced polyesters, as conventional plastics. The study explores the genetic and metabolic engineering of PHA-producing organisms for commercial PHA production.
- Authors: Lara L. Madison, G. Huisman (1999).
- Journal: Microbiology and Molecular Biology Reviews.
- Read more.
Phytozome A Comparative Platform for Green Plant Genomics
:
- Summary: This paper describes Phytozome, a comparative hub for plant genome and gene family data and analysis, providing a view of the evolutionary history of every plant gene.
- Authors: D. Goodstein et al. (2011).
- Journal: Nucleic Acids Research.
- Read more.
Phytome A Platform for Plant Comparative Genomics
:
- Summary: Phytome is an online resource for comparative genomics, providing data on protein sequences, family assignments, and functional annotations across various plant taxa.
- Authors: S. Hartmann et al. (2005).
- Journal: Nucleic Acids Research.
- Read more.
Phonon Counting and Intensity Interferometry of a Nanomechanical Resonator :
- Summary: This research explores the quantum properties of macroscopic mechanical objects using optical probes and single-photon detection, focusing on a silicon nanomechanical resonator.
- Authors: Justin D. Cohen et al. (2014).
- Journal: Nature.
- Read more.
Anisole as a Solvent for Organic Electrochemistry :
- Summary: The paper investigates the use of anisole (PhOMe) as a solvent for electrochemistry of various π-electron systems, highlighting its benefits over other solvents.
- Authors: J. Jaworski et al. (2005).
- Journal: Journal of Electroanalytical Chemistry.
- Read more.
Phylomemetic Patterns in Science Evolution – The Rise and Fall of Scientific Fields :
- Summary: This paper introduces a method for reconstructing the cognitive evolution of science using big data from digital libraries, modeled as lineage relationships between scientific fields.
- Authors: David Chavalarias, Jean-Philippe Cointet (2013).
- Journal: PLoS ONE.
- Read more.
The PhenX Toolkit Get the Most From Your Measures
:
- Summary: This paper discusses the PhenX project, aimed at identifying high-priority measures for research domains to facilitate replication and validation across studies.
- Authors: Carol M. Hamilton et al. (2011).
- Journal: American Journal of Epidemiology.
- Read more.
Propriétés
IUPAC Name |
[cyano-(6-methoxynaphthalen-2-yl)methyl] 2-(3-phenyloxiran-2-yl)acetate |
Source
|
---|---|---|
Source | PubChem | |
URL | https://pubchem.ncbi.nlm.nih.gov | |
Description | Data deposited in or computed by PubChem | |
InChI |
InChI=1S/C23H19NO4/c1-26-19-10-9-16-11-18(8-7-17(16)12-19)21(14-24)27-22(25)13-20-23(28-20)15-5-3-2-4-6-15/h2-12,20-21,23H,13H2,1H3 |
Source
|
Source | PubChem | |
URL | https://pubchem.ncbi.nlm.nih.gov | |
Description | Data deposited in or computed by PubChem | |
InChI Key |
NZOVBSWOWQLAJG-UHFFFAOYSA-N |
Source
|
Source | PubChem | |
URL | https://pubchem.ncbi.nlm.nih.gov | |
Description | Data deposited in or computed by PubChem | |
Canonical SMILES |
COC1=CC2=C(C=C1)C=C(C=C2)C(C#N)OC(=O)CC3C(O3)C4=CC=CC=C4 |
Source
|
Source | PubChem | |
URL | https://pubchem.ncbi.nlm.nih.gov | |
Description | Data deposited in or computed by PubChem | |
Molecular Formula |
C23H19NO4 |
Source
|
Source | PubChem | |
URL | https://pubchem.ncbi.nlm.nih.gov | |
Description | Data deposited in or computed by PubChem | |
Molecular Weight |
373.4 g/mol |
Source
|
Source | PubChem | |
URL | https://pubchem.ncbi.nlm.nih.gov | |
Description | Data deposited in or computed by PubChem | |
Q & A
Q1: Does the presence of a glucosyl group affect the interaction of phenolic compounds with PVP?
A2: Yes, the presence of a glucosyl group significantly hinders the interaction between phenolic compounds and PVP in aqueous solutions. [] This effect is observed with both phenyl glucoside (PhOGl) and hydroxyphenyl glucoside (HOPhOGl). [] The glucosyl group likely disrupts the hydrophobic interactions and hydrogen bonding between the phenolic compounds and PVP. []
Q2: How does the acidity of phenolic compounds influence their interaction with PVP?
A3: The acidity of phenolic compounds plays a crucial role in their interaction with PVP. More acidic phenols, such as 4-hydroxy-3-nitrophenol (HOPhNO2), tend to exhibit stronger binding to PVP and can even precipitate the polymer at lower concentrations compared to less acidic phenols. [] This suggests a significant contribution of hydrogen bonding from the phenolic hydroxyl group in the binding process. []
Q3: What are the spectroscopic characteristics of phenyl methyl ether (PhOMe)?
A3: Phenyl methyl ether (this compound), also known as anisole, exhibits characteristic spectroscopic features:
Q4: What is the molecular formula and weight of phenyl methyl ether (this compound)?
A4: The molecular formula of phenyl methyl ether (this compound) is C7H8O. Its molecular weight is 108.14 g/mol.
Q5: Is phenyl methyl ether (this compound) compatible with strong oxidizing agents?
A5: Phenyl methyl ether (this compound) is generally not compatible with strong oxidizing agents. The ether functional group is susceptible to oxidation, which can lead to the formation of peroxides or other byproducts.
Q6: How does the stability of di-iron propanedithiolate complexes change with different tris(aromatic)phosphine ligands?
A8: The stability and electronic properties of di-iron propanedithiolate complexes are significantly influenced by the nature of the tris(aromatic)phosphine ligands coordinated to the iron centers. [] For instance, complexes with highly electron-rich phosphine ligands, such as tris(4-methoxyphenyl)phosphine (P(this compound-p)3), exhibit a greater red shift in CO-stretching frequencies and longer Fe-Fe bond distances compared to complexes with less electron-donating phosphines like tris(4-fluorophenyl)phosphine (P(PhF-p)3). [] This suggests that the electron-donating ability of the phosphine ligand directly affects the electronic environment of the iron centers and, consequently, the stability and reactivity of the complex. []
Q7: How do polar molecules (PMs) regulate the regio- and stereoselectivity of conjugated diene polymerization catalyzed by CGC-type rare-earth metal catalysts?
A9: Polar molecules (PMs), such as anisole (this compound), can significantly impact the regio- and stereoselectivity of conjugated diene polymerization reactions catalyzed by CGC-type rare-earth metal complexes. [] These PMs act as continuously coordinated neutral ligands to the cationic active species generated in the reaction. [] The coordination of PMs influences the steric and electronic environment around the metal center, leading to changes in the orientation and insertion of the diene monomers during polymerization. [] For instance, the addition of PMs like this compound can switch the selectivity of isoprene (IP) polymerization from a mixture of isomers to highly selective 3,4-polymerization (up to 99% selectivity). [] This control over regio- and stereoselectivity through PM addition offers a simple and effective method to tune the properties of the resulting polymers. []
Q8: Can you explain the role of phenyl methyl ether (this compound) in cobalt-catalyzed ring-opening addition reactions?
A10: Phenyl methyl ether (this compound) serves as a solvent in cobalt-catalyzed ring-opening additions of azabenzonorbornadienes. [] The reaction, catalyzed by [Cp*CoI2(CO)] in the presence of AgSbF6 and Fe(OAc)2, proceeds via C(sp3)-H bond activation of 8-methylquinoline. [] While the specific role of this compound is not explicitly detailed in the research, it likely facilitates the formation and stabilization of reactive intermediates, influencing reaction rates and yields.
Q9: How do DFT calculations explain the role of polar molecules (PMs) in regulating polymerization selectivity?
A11: Density functional theory (DFT) calculations provide insights into the mechanism by which PMs influence polymerization selectivity. These calculations reveal that PMs, by coordinating to the metal center of the catalyst, alter the energy landscape of the reaction intermediates and transition states involved in monomer insertion. [] This, in turn, affects the relative energies of different reaction pathways, leading to preferential formation of specific regio- and stereoisomers of the polymer. []
Q10: Have there been computational studies on the intramolecular stabilization of [FeFe]-hydrogenase mimics?
A12: Yes, computational studies employing density functional theory (DFT) have been conducted to investigate the intramolecular stabilization of [FeFe]-hydrogenase mimics. [] One study focused on a mono-substituted complex, [Fe2(CO)5(μ-naphthalene-2-thiolate)2(P(this compound-p)3)], which incorporates structural features found in both [NiFe] and [FeFe]-hydrogenase enzymes. [] DFT calculations revealed that π-π stacking interactions between a naphthyl moiety and the terminal bulky phosphine ligand contribute significantly to the stability of the reduced and protonated forms of the complex during electrocatalytic proton reduction. [] This interaction hinders rotation of the phosphine ligand and helps maintain a favorable geometry for proton reduction. [] These findings provide valuable insights into the design of more efficient and stable biomimetic catalysts for hydrogen evolution. []
Q11: How does the substitution pattern on the phenyl ring of phenyl methyl ether (this compound) derivatives affect their activity as cholesterol absorption inhibitors?
A13: Studies on carboxy-substituted 2-azetidinones revealed that replacing a methoxy group (this compound) at the C-4 position with a phenyl ring linked to a carboxylic acid ester (Ph-linker-COOR) significantly enhanced cholesterol absorption inhibition. [] This suggests that the presence of a carboxylic acid functionality, rather than a methoxy group, at that specific position is crucial for increased activity. [] These findings highlight the importance of SAR studies in optimizing the potency of drug candidates.
Q12: How does introducing electron-donating or electron-withdrawing groups on the β-ketiminato ligand affect the catalytic activity of neutral nickel(II) olefin polymerization catalysts?
A14: The catalytic activity, molecular weight (MW) and branching of polyethylene produced by neutral nickel(II) olefin polymerization catalysts are significantly influenced by the electronic properties of the β-ketiminato ligand. [] Electron-deficient ligands were found to enhance catalytic activity, decrease MW, and increase branching in the resulting polyethylene. [] Conversely, electron-rich ligands generally lead to lower activity, higher MW, and less branched polymers. [] This suggests that the electron density at the nickel center, modulated by the ligand, plays a crucial role in determining the catalyst's performance. []
Q13: How do substituents on the thieno[3,2-b]thiophene (TT) core affect the fluorescence properties of triphenylamine (TPA) and 4,4'-dimethoxytriphenylamine (TPA(OMe)2)-functionalized TT fluorophores?
A15: The introduction of various electron-donating and electron-withdrawing substituents on the TT core significantly impacts the photophysical properties of TPA and TPA(OMe)2-functionalized TT fluorophores. [] The nature of the substituent influences the electron density distribution within the molecule, affecting the energy levels of the highest occupied molecular orbital (HOMO) and the lowest unoccupied molecular orbital (LUMO). [] This, in turn, impacts the energy gap between the HOMO and LUMO, which directly correlates with the wavelength of light absorbed and emitted by the fluorophore. [] As a result, the emission color of these fluorophores can be finely tuned from orange to dark blue by varying the substituents on the TT core. []
Avertissement et informations sur les produits de recherche in vitro
Veuillez noter que tous les articles et informations sur les produits présentés sur BenchChem sont destinés uniquement à des fins informatives. Les produits disponibles à l'achat sur BenchChem sont spécifiquement conçus pour des études in vitro, qui sont réalisées en dehors des organismes vivants. Les études in vitro, dérivées du terme latin "in verre", impliquent des expériences réalisées dans des environnements de laboratoire contrôlés à l'aide de cellules ou de tissus. Il est important de noter que ces produits ne sont pas classés comme médicaments et n'ont pas reçu l'approbation de la FDA pour la prévention, le traitement ou la guérison de toute condition médicale, affection ou maladie. Nous devons souligner que toute forme d'introduction corporelle de ces produits chez les humains ou les animaux est strictement interdite par la loi. Il est essentiel de respecter ces directives pour assurer la conformité aux normes légales et éthiques en matière de recherche et d'expérimentation.