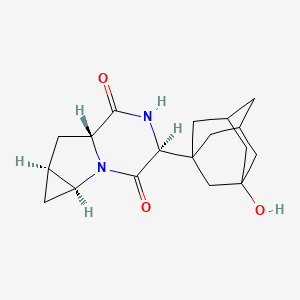
Saxagliptin Cyclic Amide
Vue d'ensemble
Description
Saxagliptin Cyclic Amide (SCA), also referred to as saxagliptin cyclic amidine, is a key degradation product and metabolite of saxagliptin, a dipeptidyl peptidase-4 (DPP-4) inhibitor used in type 2 diabetes therapy. Structurally, SCA arises from the intramolecular cyclization of saxagliptin’s free base, forming a stable 6-membered cyclic amidine ring (Figure 1) . This cyclization occurs spontaneously under specific conditions, particularly during synthesis or storage, and is influenced by environmental factors such as temperature, humidity, and excipient composition . SCA has been identified in both human and rat plasma, alongside other metabolites like 5-hydroxysaxagliptin and saxagliptin–cysteine conjugates, confirming its relevance in pharmacokinetic and stability studies .
The formation of SCA is a critical quality attribute in saxagliptin formulations, as excessive levels may compromise drug efficacy and safety. Regulatory guidelines emphasize controlling SCA levels through optimized manufacturing processes and excipient selection .
Méthodes De Préparation
Synthetic Routes and Reaction Conditions: The preparation of saxagliptin involves the coupling of N-Boc-3-hydroxyadamantylglycine with methanoprolineamide using EDC as a coupling agent . The formation of saxagliptin cyclic amide occurs as a degradation product under specific conditions, such as high relative humidity and the presence of reactive impurities like polyethylene glycol degradation products .
Industrial Production Methods: Industrial production of saxagliptin typically involves enzyme-catalyzed reactions. For instance, the biocatalytic semi-synthesis of saxagliptin involves the use of phenylalanine dehydrogenase and formate dehydrogenase to convert 2-(3-hydroxy-1-adamantyl)-2-oxoacetic acid into (S)-3-hydroxyadamantane glycine . The process is optimized for high yield and efficiency, making it suitable for large-scale production.
Analyse Des Réactions Chimiques
Formation
Saxagliptin can degrade into Saxagliptin Cyclic Amide. The synthesis of saxagliptin can be achieved through amide coupling of N-Boc-3-hydroxyadamantylglycine and methanoprolineamide with EDC .
Degradation Products
Key degradation products of Saxagliptin include cyclic amidine, epimer, and formyl amide .
Chemical Reactions
This compound undergoes various chemical reactions:
- Hydrolysis Hydrolysis leads to the formation of saxagliptin formyl amide impurity.
- Oxidation Oxidation results in the formation of 5-hydroxysaxagliptin.
- Substitution Substitution involves the reaction with nucleophiles like L-cysteine to form thiazolinic acid metabolites. L-cysteine decreases the stability of saxagliptin, which binds to cysteine in a nonenzymatic manner. The nitrile cyanopyrrolidine moiety in the saxagliptin structure is an essential functional group for the interaction with L-cysteine .
Reactivity with L-Cysteine
L-cysteine decreases the stability of saxagliptin in the absence of an enzyme source, which indicates that saxagliptin binds to cysteine in a nonenzymatic manner. The nitrile cyanopyrrolidine moiety in the saxagliptin structure is an essential functional group for the interaction with L-cysteine . This covalent binding of saxagliptin is an irreversible reaction that forms a thiazoline ring before deamination .
Metabolites
Observed metabolic pathways of saxagliptin include hydroxylation and conjugation with cysteine, glutathione, sulfate, and glucuronide. Saxagliptin can covalently bind to the thiol groups of cysteine residues of endogenous proteins in vivo, indicating the potential for saxagliptin to cause drug-induced liver injury .
Table of Saxagliptin Metabolites
No. | Metabolites | tR (min) | Theoretical $$M+H]+ | Measured $$M+H]+ | Mass Error (ppm) | Reaction Type |
---|---|---|---|---|---|---|
P | Saxagliptin | 5.3 | 316.2020 | 316.2017 | -0.95 | |
M1 | Saxagliptin cyclic amidine | 4.9 | 316.2020 | 316.2017 | -0.95 | cyclization |
M2 | 5-Hydroxysaxagliptin | 4.2 | 332.1969 | 332.1965 | -1.2 | hydroxylation |
M3 | Saxagliptin–cysteine conjugate | 4.4 | 420.1952 | 420.1931 | -4.99 | cysteine conjugation |
M4 | 5-Hydroxysaxagliptin–cysteine conjugate | 3.5 | 436.1901 | 436.1895 | -1.40 | Hydroxylation and cysteine conjugation |
M5 | Saxagliptin–cysteinylglycine conjugate | 4.7 | 477.2166 | 477.2163 | -0.63 | glutathione conjugation |
Applications De Recherche Scientifique
Chemistry
- Degradation Pathways : Research has focused on the degradation pathways and stability of saxagliptin formulations. Understanding these pathways helps in optimizing drug formulations to enhance shelf life and efficacy.
- Analytical Techniques : High-performance liquid chromatography coupled with mass spectrometry (HPLC-MS) has been employed to study saxagliptin's forced degradation products, providing insights into its stability under various conditions .
Biology
- Metabolic Pathways : Studies have investigated the metabolic pathways of saxagliptin and its cyclic amide form, revealing interactions with cytochrome P450 isoforms which are critical for drug metabolism .
- Toxicological Effects : Research has highlighted potential toxicological effects related to saxagliptin's metabolites, particularly concerning drug-induced liver injury due to covalent binding with thiol groups in proteins .
Medicine
- Clinical Efficacy : Clinical studies have demonstrated the efficacy of saxagliptin in improving glycemic control in patients with type 2 diabetes. Its unique properties allow it to maintain prolonged binding to DPP-4, resulting in sustained therapeutic effects .
- Safety Profiles : Investigations into the safety profiles of saxagliptin and its cyclic amide have emphasized the importance of understanding impurities and their impact on drug safety and efficacy .
Case Studies
Several case studies have illustrated the applications of saxagliptin cyclic amide in clinical settings:
- Clinical Trials : A study involving patients with type 2 diabetes demonstrated that saxagliptin significantly improved glycemic control compared to placebo over a 24-week period, highlighting its effectiveness as a therapeutic agent .
- Metabolite Analysis : Research on the metabolites of saxagliptin revealed that certain metabolites could potentially lead to adverse effects, necessitating further exploration into their pharmacokinetics and safety profiles .
- Drug Interaction Studies : Studies assessing interactions between saxagliptin and other medications indicated that co-administration with drugs affecting cytochrome P450 enzymes could alter its metabolism, emphasizing the need for careful monitoring during treatment.
Mécanisme D'action
Saxagliptin Cyclic Amide, like saxagliptin, inhibits dipeptidyl peptidase-4 (DPP-4). DPP-4 is responsible for degrading incretin hormones such as glucagon-like peptide-1 (GLP-1) and glucose-dependent insulinotropic polypeptide (GIP). Inhibition of DPP-4 increases the levels of these hormones, enhancing insulin secretion and reducing blood glucose levels . Saxagliptin forms a reversible, histidine-assisted covalent bond with the S630 hydroxyl oxygen on DPP-4 .
Comparaison Avec Des Composés Similaires
Comparison with Structural Analogs and Metabolites
Saxagliptin Metabolites and Degradants
Saxagliptin undergoes complex metabolic and degradation pathways, generating several structurally related compounds (Table 1):
Table 1. Structural and functional comparison of saxagliptin-related compounds.
SCA is distinguished by its cyclic structure, which confers greater stability compared to linear metabolites like 5-hydroxysaxagliptin. However, SCA lacks pharmacological activity, unlike 5-hydroxysaxagliptin, which retains ~50% of saxagliptin’s DPP-4 inhibitory potency .
Epimeric Impurities
SCA’s epimer, epi-cyclic amidine (ESCA), forms under specific formulation conditions. ESCA and SCA differ in stereochemistry at the cyclization site, leading to distinct chromatographic retention times and mass spectra . The ratio of SCA to ESCA is highly sensitive to excipient composition, particularly polyethylene glycol (PEG) content, which alters reaction kinetics during film-coating processes .
Stability and Degradation Pathways
Environmental and Formulation Influences
Stress stability studies reveal that SCA formation dominates in solid-state saxagliptin under high humidity and temperature (Table 2) :
Condition | Primary Impurities | Relative Abundance |
---|---|---|
High humidity (75% RH, 40°C) | SCA | 70–80% |
PEG-rich film coatings | SCA, ESCA, SFA | SCA:ESCA:SFA ≈ 5:3:2 |
Acidic pH | 5-Hydroxysaxagliptin | <10% |
Table 2. Degradation impurities under varying stress conditions.
The solvent’s proton affinity and autoprotolysis constant further influence cyclization rates. For example, polar aprotic solvents accelerate SCA formation due to enhanced nucleophilicity of the amine group .
Comparison with Other DPP-4 Inhibitors
Unlike saxagliptin, other DPP-4 inhibitors like vildagliptin and anagliptin undergo hydrolytic metabolism mediated by DPP enzymes, yielding carboxylic acid metabolites. In contrast, saxagliptin’s amide intermediate cannot be hydrolyzed by DPP-4 or DPP-8, resulting in a unique metabolic profile dominated by oxidative pathways (e.g., 5-hydroxysaxagliptin) rather than hydrolysis .
Metabolic and Pharmacokinetic Considerations
Impurity Profiles and Control Strategies
The European Medicines Agency (EMA) mandates strict control of SCA levels in saxagliptin formulations. Strategies include:
Activité Biologique
Saxagliptin Cyclic Amide is a derivative of saxagliptin, a potent dipeptidyl peptidase-4 (DPP-4) inhibitor widely used in the management of type 2 diabetes mellitus (T2DM). This article delves into the biological activity of this compound, including its mechanism of action, pharmacokinetics, and various research findings.
Target Enzyme: Dipeptidyl Peptidase-4 (DPP-4)
this compound primarily inhibits the DPP-4 enzyme, which plays a crucial role in the degradation of incretin hormones, such as glucagon-like peptide-1 (GLP-1) and glucose-dependent insulinotropic polypeptide (GIP). By inhibiting DPP-4, saxagliptin increases the levels of active GLP-1 and GIP, enhancing glucose-dependent insulin secretion and improving blood glucose regulation .
Covalent Bond Formation
Saxagliptin forms a reversible, histidine-assisted covalent bond with the S630 hydroxyl oxygen on DPP-4 through its nitrile group. This interaction is essential for its inhibitory activity .
Pharmacokinetics
Saxagliptin is characterized by its favorable pharmacokinetic profile:
- Absorption : Orally absorbed with a bioavailability of approximately 67% .
- Peak Concentration (Tmax) : Achieved within 2 hours for saxagliptin and 4 hours for its active metabolite BMS-510849 .
- Half-Life (t1/2) : Ranges from 2.2 to 3.8 hours for saxagliptin .
- Metabolism : Primarily mediated by cytochrome P450 3A4/5, producing an active metabolite with reduced potency .
Clinical Studies
Numerous clinical studies have demonstrated the efficacy and safety of saxagliptin in managing T2DM. For instance:
- A double-blind study involving 40 patients showed that saxagliptin significantly reduced HbA1c levels compared to placebo .
- Another study highlighted saxagliptin's nephroprotective effects alongside its anti-inflammatory properties, contributing to improved metabolic outcomes in diabetic patients .
Case Studies
Research has also identified potential adverse effects associated with saxagliptin:
- A case report documented drug-induced liver injury in a patient taking saxagliptin combined with metformin. This underscores the importance of monitoring liver function in patients receiving this medication .
- The formation of thiazoline-containing thiol metabolites was observed in male rats administered saxagliptin, indicating possible interactions with endogenous proteins that could lead to toxicity .
Comparative Analysis
This compound can be compared with other DPP-4 inhibitors based on their potency and selectivity:
Compound | DPP-4 Inhibition Potency | Unique Features |
---|---|---|
Saxagliptin | 1.3 nM | Cyanopyrrolidine structure; reversible inhibition |
Sitagliptin | 18 nM | Less potent than saxagliptin |
Vildagliptin | 13 nM | Similar structure but different metabolic pathways |
Linagliptin | Not directly comparable | Does not form reactive metabolites |
Q & A
Basic Research Questions
Q. What analytical techniques are recommended for characterizing the cyclic amide moiety in Saxagliptin to ensure structural integrity and purity?
- Methodological Answer :
- Nuclear Magnetic Resonance (NMR) : Use - and -NMR to confirm the cyclic amide structure, focusing on chemical shifts for the amide carbonyl (160–180 ppm in -NMR) and adjacent protons (δ 3.0–4.5 ppm in -NMR). Compare with synthetic intermediates described in patent literature .
- High-Performance Liquid Chromatography (HPLC) : Employ reverse-phase HPLC with UV detection (λ = 210–230 nm) to assess purity (>98% by area). Use a C18 column and isocratic elution (acetonitrile/water with 0.1% TFA) .
- X-ray Crystallography : For unambiguous structural confirmation, crystallize the compound and analyze diffraction patterns, referencing published crystal structures of DPP-4 inhibitors .
Q. How can researchers ensure reproducibility in synthesizing Saxagliptin cyclic amide intermediates?
- Methodological Answer :
- Detailed Experimental Protocols : Document reaction parameters (temperature, solvent, catalyst loading) and purification steps (e.g., recrystallization solvents, column chromatography gradients). For example, the patent by Application No. 1229/MUMNP/2013 specifies a cyclization step using THF and NaH at 0–5°C .
- Batch-to-Batch Consistency : Report yields, melting points, and spectroscopic data for each batch. Include supplementary materials with raw NMR and HPLC traces .
- Reference Standards : Use commercially available Saxagliptin (e.g., Sigma-Aldrich T6203) as a control for comparative analysis .
Advanced Research Questions
Q. What strategies resolve discrepancies in DPP-4 inhibition potency between this compound and its analogs?
- Methodological Answer :
- Comparative Biochemical Assays : Conduct dose-response curves (0.1–100 nM) using recombinant human DPP-4. Calculate IC values and compare with literature data (e.g., Saxagliptin’s Ki = 0.6–1.3 nM ).
- Structural-Activity Relationship (SAR) Analysis : Correlate inhibitory activity with substituent effects on the cyclic amide. Use molecular docking (e.g., AutoDock Vina) to model interactions with DPP-4’s active site .
- Statistical Validation : Apply ANOVA to assess significance across replicates and batches, addressing outliers through rigorous data filtering .
Q. How should researchers design experiments to optimize the cyclization step in Saxagliptin amide synthesis?
- Methodological Answer :
- Design of Experiments (DoE) : Vary parameters like temperature (0–25°C), catalyst (NaH vs. KCO), and solvent polarity (THF vs. DMF). Use response surface methodology to identify optimal conditions .
- Real-Time Monitoring : Employ in-situ FTIR or HPLC to track reaction progress and intermediate formation. Terminate reactions at >95% conversion to minimize byproducts .
- Scale-Up Considerations : Assess feasibility for gram-scale synthesis by testing agitation rates and heat transfer efficiency in pilot reactors .
Q. Data Analysis and Interpretation
Q. What methodologies assess the stability of Saxagliptin’s cyclic amide under physiological conditions?
- Methodological Answer :
- Accelerated Stability Studies : Incubate Saxagliptin in buffers (pH 1.2, 4.5, 7.4) at 37°C. Sample aliquots at 0, 1, 3, 7 days and analyze via HPLC for degradation products (e.g., open-chain amide derivatives) .
- Mass Spectrometry (LC-MS) : Identify degradation pathways by detecting fragments (e.g., m/z 243.1 for hydrolyzed amide) .
- Kinetic Modeling : Calculate degradation rate constants (k) and half-life (t) using first-order kinetics .
Q. Literature and Synthesis Review
Q. How can researchers systematically compare synthetic routes for this compound derivatives?
- Methodological Answer :
- Database Mining : Use SciFinder to compile patents and journal articles (e.g., keyword: “Saxagliptin AND cyclization”). Filter by yield (>70%) and scalability (gram-scale) .
- Cost-Benefit Analysis : Tabulate reagents, catalysts, and reaction times for each route. Prioritize methods with low Pd catalyst loading to reduce costs .
- Green Chemistry Metrics : Calculate E-factors (kg waste/kg product) and atom economy for sustainability assessment .
Propriétés
IUPAC Name |
(2S,4S,6R,9S)-9-(3-hydroxy-1-adamantyl)-1,8-diazatricyclo[4.4.0.02,4]decane-7,10-dione | |
---|---|---|
Source | PubChem | |
URL | https://pubchem.ncbi.nlm.nih.gov | |
Description | Data deposited in or computed by PubChem | |
InChI |
InChI=1S/C18H24N2O3/c21-15-13-3-11-2-12(11)20(13)16(22)14(19-15)17-4-9-1-10(5-17)7-18(23,6-9)8-17/h9-14,23H,1-8H2,(H,19,21)/t9?,10?,11-,12-,13+,14+,17?,18?/m0/s1 | |
Source | PubChem | |
URL | https://pubchem.ncbi.nlm.nih.gov | |
Description | Data deposited in or computed by PubChem | |
InChI Key |
ZHBXKHDARSBKNT-YGADWWLDSA-N | |
Source | PubChem | |
URL | https://pubchem.ncbi.nlm.nih.gov | |
Description | Data deposited in or computed by PubChem | |
Canonical SMILES |
C1C2C1N3C(C2)C(=O)NC(C3=O)C45CC6CC(C4)CC(C6)(C5)O | |
Source | PubChem | |
URL | https://pubchem.ncbi.nlm.nih.gov | |
Description | Data deposited in or computed by PubChem | |
Isomeric SMILES |
C1[C@@H]2[C@H]1N3[C@H](C2)C(=O)N[C@H](C3=O)C45CC6CC(C4)CC(C6)(C5)O | |
Source | PubChem | |
URL | https://pubchem.ncbi.nlm.nih.gov | |
Description | Data deposited in or computed by PubChem | |
Molecular Formula |
C18H24N2O3 | |
Source | PubChem | |
URL | https://pubchem.ncbi.nlm.nih.gov | |
Description | Data deposited in or computed by PubChem | |
Molecular Weight |
316.4 g/mol | |
Source | PubChem | |
URL | https://pubchem.ncbi.nlm.nih.gov | |
Description | Data deposited in or computed by PubChem | |
Avertissement et informations sur les produits de recherche in vitro
Veuillez noter que tous les articles et informations sur les produits présentés sur BenchChem sont destinés uniquement à des fins informatives. Les produits disponibles à l'achat sur BenchChem sont spécifiquement conçus pour des études in vitro, qui sont réalisées en dehors des organismes vivants. Les études in vitro, dérivées du terme latin "in verre", impliquent des expériences réalisées dans des environnements de laboratoire contrôlés à l'aide de cellules ou de tissus. Il est important de noter que ces produits ne sont pas classés comme médicaments et n'ont pas reçu l'approbation de la FDA pour la prévention, le traitement ou la guérison de toute condition médicale, affection ou maladie. Nous devons souligner que toute forme d'introduction corporelle de ces produits chez les humains ou les animaux est strictement interdite par la loi. Il est essentiel de respecter ces directives pour assurer la conformité aux normes légales et éthiques en matière de recherche et d'expérimentation.