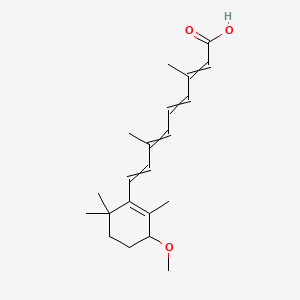
(2E,4E,6E,8E)-9-(3-Methoxy-2,6,6-trimethylcyclohex-1-EN-1-YL)-3,7-dimethylnona-2,4,6,8-tetraenoic acid
- Cliquez sur DEMANDE RAPIDE pour recevoir un devis de notre équipe d'experts.
- Avec des produits de qualité à un prix COMPÉTITIF, vous pouvez vous concentrer davantage sur votre recherche.
Vue d'ensemble
Description
4-Methoxy Retinoic Acid, also known as (±)-4-Methoxyretinoic Acid, is an impurity contained in samples of all trans-retinoic acid . It has a molecular weight of 330.46 and a molecular formula of C21H30O3 . It is structurally related to vitamin A and is considered an active metabolite of retinol .
Molecular Structure Analysis
The IUPAC name for 4-Methoxy Retinoic Acid is (2 E ,4 E ,6 E ,8 E )-9- (3-methoxy-2,6,6-trimethylcyclohexen-1-yl)-3,7-dimethylnona-2,4,6,8-tetraenoic acid . The InChI and SMILES strings provide a detailed description of the molecule’s structure .
Applications De Recherche Scientifique
Antitumor Activity of Retinoids
Research has demonstrated that retinoids, including 4-methoxy retinoic acid, play a crucial role in cancer therapy. Retinoids induce differentiation and/or growth inhibition in various tumor cell lines. Their effects are mediated through changes in gene expression via specific nuclear receptors, notably retinoic acid receptors (RARs). Clinical studies have observed significant responses to retinoid treatments in patients with acute promyelocytic leukemia (APL), cutaneous T-cell malignancies, and other dermatologic cancers. The combination of 13-cis-retinoic acid with interferon alpha has also produced high objective response rates in patients with squamous cell carcinomas. This underscores the need for targeted clinical trials to define the spectrum of tumors responsive to retinoid-based treatments and to further understand the biological mechanisms associated with therapeutic responses (Smith et al., 1992).
Cancer Preventive Potential of Retinoids
The International Agency for Research on Cancer (IARC) evaluated the cancer preventive potential of several retinoids. While some retinoids were deemed unsuitable for chemoprevention due to toxicity or ineffectiveness, others showed promise. For instance, 9-cis-retinoic acid displayed potential but required further data for confirmation. This comprehensive review underscores the nuanced role of retinoids in cancer prevention, highlighting the need for additional research to clarify their efficacy and safety profiles (Miller et al., 2000).
Retinoids in Dermatology and Acne Treatment
Retinoids have been extensively studied for their role in treating acne through mechanisms such as modulating gene expression in sebocytes, reducing inflammation, and affecting sebum production. The review of 13-cis retinoic acid's conversion to all-trans retinoic acid and its impact on acne-related inflammation underscores the potential of retinoids in dermatological applications beyond their traditional use, offering insights into future acne therapies (Zouboulis, 2001).
Role in Reproductive Technology
Retinoic acid supplementation during in vitro oocyte maturation has shown promise in enhancing embryo development in livestock. The addition of retinoic acid to oocyte maturation media improves various developmental metrics, such as nuclear oocyte maturation rates and embryo development, due to its antioxidant properties and anti-apoptotic effects. This suggests retinoic acid's potential utility in improving assisted reproductive technologies across different species (Abdelnour et al., 2019).
Mécanisme D'action
Target of Action
4-Methoxy Retinoic Acid, like other retinoids, primarily targets the Retinoic Acid Receptors (RARs) . These receptors are nuclear receptors that play a critical role in regulating cellular proliferation, development, and differentiation in response to endogenous retinoids .
Mode of Action
4-Methoxy Retinoic Acid interacts with its targets, the RARs, by binding to them . This binding leads to a conformational change in the RARs, allowing the release of corepressors and recruitment of coactivators . This results in the transcriptional activation of target genes via specific Retinoic Acid Response Elements .
Biochemical Pathways
The biochemical pathways affected by 4-Methoxy Retinoic Acid involve the metabolism of Vitamin A to Retinoic Acid (RA) and further oxidation of RA . The enzymes that metabolize Vitamin A to RA and the cytochrome P450 Cyp26 family of enzymes that further oxidize RA are key components of these pathways .
Pharmacokinetics
ATRA is absorbed rapidly into plasma after oral dosing and exhibits a terminal half-life of 13-16 hours . The major metabolites of ATRA in humans are 4-hydroxy- and 4-oxo-retinoic acid .
Result of Action
The molecular and cellular effects of 4-Methoxy Retinoic Acid’s action involve the regulation of cell differentiation, proliferation, and apoptosis . It also plays a role in the maintenance of hematopoietic stem cell dormancy and the regulation of the expression of Hox genes, which largely affect normal morphogenesis in vertebrates .
Action Environment
Environmental factors can influence the action, efficacy, and stability of 4-Methoxy Retinoic Acid. For instance, the occurrence of xenobiotic environmental pollutants that interfere with RARs and disrupt the RAR signaling may pose a threat to the health of wild animals and humans . Additionally, climate change and population growth can exacerbate the environmental risks of retinoid signaling disruption .
Safety and Hazards
Orientations Futures
Retinoic acids, including 4-Methoxy Retinoic Acid, have been investigated extensively for their utility in cancer prevention and treatment. Success has been achieved with their use in the treatment of subtypes of leukemia harboring chromosomal translocations . Promising results have been observed in the breast cancer prevention setting, where fenretinide prevention trials have provided a strong rationale for further investigation in young women at high-risk for breast cancer . Ongoing phase 3 randomized trials investigating retinoids in combination with chemotherapy in non-small cell lung cancer aim to definitively characterize the role of retinoids in this tumor type .
Analyse Biochimique
Biochemical Properties
4-Methoxy Retinoic Acid interacts with various enzymes, proteins, and other biomolecules. It is involved in retinoid-converting enzymes in animals and bacteria, including retinol dehydrogenase, alcohol dehydrogenase, aldo-keto reductase, and aldehyde dehydrogenase .
Cellular Effects
4-Methoxy Retinoic Acid has significant effects on various types of cells and cellular processes. It is known to influence cell function, including impacts on cell signaling pathways, gene expression, and cellular metabolism .
Molecular Mechanism
The molecular mechanism of 4-Methoxy Retinoic Acid involves its interactions with biomolecules, enzyme inhibition or activation, and changes in gene expression. The discovery of a nuclear retinoic acid receptor (RAR), which functions as ligand-inducible transcription factors, was a breakthrough in understanding how retinoids exert their pleiotropic effects .
Temporal Effects in Laboratory Settings
The effects of 4-Methoxy Retinoic Acid change over time in laboratory settings. High retinoic acid (RA) signaling early was sufficient to promote M cone fate and suppress L cone fate in retinal organoids .
Metabolic Pathways
4-Methoxy Retinoic Acid is involved in the metabolic pathways of vitamin A. Vitamin A is metabolized to retinoic acid and then to 4-OH and 4-oxoRA .
Subcellular Localization
Retinoic acid receptors (RARs), which 4-Methoxy Retinoic Acid may interact with, have been found to exhibit different intracellular localization patterns depending on culture conditions .
Propriétés
{ "Design of the Synthesis Pathway": "The synthesis of 4-Methoxy Retinoic Acid can be achieved by starting with commercially available starting materials and using a series of chemical reactions to introduce the required functional groups.", "Starting Materials": [ "4-methoxybenzaldehyde", "ethyl acetoacetate", "sodium ethoxide", "acetic anhydride", "pyridine", "chromic acid", "methanol", "sodium hydroxide", "retinol" ], "Reaction": [ "Step 1: Condensation of 4-methoxybenzaldehyde and ethyl acetoacetate in the presence of sodium ethoxide to form ethyl 4-methoxy-3-oxo-3-phenylpropanoate.", "Step 2: Hydrolysis of the ester using sodium hydroxide to give 4-methoxyphenyl-3-oxo-4-phenylbutanoic acid.", "Step 3: Conversion of the acid to its anhydride using acetic anhydride and pyridine.", "Step 4: Treatment of the anhydride with chromic acid in methanol to form 4-methoxyretinaldehyde.", "Step 5: Reduction of 4-methoxyretinaldehyde to 4-methoxyretinol using sodium borohydride.", "Step 6: Oxidation of 4-methoxyretinol to 4-Methoxy Retinoic Acid using chromic acid." ] } | |
Numéro CAS |
81121-20-2 |
Formule moléculaire |
C21H30O3 |
Poids moléculaire |
330.5 g/mol |
Nom IUPAC |
(2E,4E,6E,8E)-9-(3-methoxy-2,6,6-trimethylcyclohexen-1-yl)-3,7-dimethylnona-2,4,6,8-tetraenoic acid |
InChI |
InChI=1S/C21H30O3/c1-15(8-7-9-16(2)14-20(22)23)10-11-18-17(3)19(24-6)12-13-21(18,4)5/h7-11,14,19H,12-13H2,1-6H3,(H,22,23)/b9-7+,11-10+,15-8+,16-14+ |
Clé InChI |
KZKUSKHEEFFSTO-XPSLGPGOSA-N |
SMILES isomérique |
CC1=C(C(CCC1OC)(C)C)/C=C/C(=C/C=C/C(=C/C(=O)O)/C)/C |
SMILES |
CC1=C(C(CCC1OC)(C)C)C=CC(=CC=CC(=CC(=O)O)C)C |
SMILES canonique |
CC1=C(C(CCC1OC)(C)C)C=CC(=CC=CC(=CC(=O)O)C)C |
Synonymes |
(+/-)-4-Methoxyretinoic Acid; |
Origine du produit |
United States |
Avertissement et informations sur les produits de recherche in vitro
Veuillez noter que tous les articles et informations sur les produits présentés sur BenchChem sont destinés uniquement à des fins informatives. Les produits disponibles à l'achat sur BenchChem sont spécifiquement conçus pour des études in vitro, qui sont réalisées en dehors des organismes vivants. Les études in vitro, dérivées du terme latin "in verre", impliquent des expériences réalisées dans des environnements de laboratoire contrôlés à l'aide de cellules ou de tissus. Il est important de noter que ces produits ne sont pas classés comme médicaments et n'ont pas reçu l'approbation de la FDA pour la prévention, le traitement ou la guérison de toute condition médicale, affection ou maladie. Nous devons souligner que toute forme d'introduction corporelle de ces produits chez les humains ou les animaux est strictement interdite par la loi. Il est essentiel de respecter ces directives pour assurer la conformité aux normes légales et éthiques en matière de recherche et d'expérimentation.