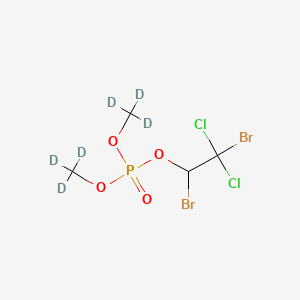
Naled-d6
- Cliquez sur DEMANDE RAPIDE pour recevoir un devis de notre équipe d'experts.
- Avec des produits de qualité à un prix COMPÉTITIF, vous pouvez vous concentrer davantage sur votre recherche.
Vue d'ensemble
Description
Naled-d6 is a labeled cholinesterase inhibitor . It is used as an insecticide and an acaricide . The molecular formula of this compound is C4HD6Br2Cl2O4P and it has a molecular weight of 386.82 .
Synthesis Analysis
While specific synthesis methods for this compound were not found, general methods for pesticide analysis involve techniques like QuEChERS with dSPE and SPE . These techniques demand time-consuming sample preparation and require the use of both LC-MS/MS and GC-MS/MS based instruments to cover the analysis for all regulated pesticides . A streamlined approach for working with LC-MS/MS featuring a dual electrospray ionization (ESI) and atmospheric pressure chemical ionization (APCI) sources using solvent extraction has been described for faster and easier sample preparation .Chemical Reactions Analysis
In the context of pesticide analysis, a LC-MS/MS system with dual ESI and APCI source was used to determine sensitivity for the analysis of 66 pesticides in hemp matrix . The unique ionization mechanism of chlorinated pesticides was elucidated using the LC-MS/MS system with an APCI source .Physical and Chemical Properties Analysis
The molecular weight of this compound is 386.82 . Its molecular formula is C4HD6Br2Cl2O4P . Further details about its physical and chemical properties were not found in the retrieved papers.Safety and Hazards
Naled-d6 is considered hazardous according to the 2012 OSHA Hazard Communication Standard . It is toxic if swallowed and harmful in contact with skin . It causes skin irritation and serious eye irritation . It is also very toxic to aquatic life . Precautions include avoiding release to the environment and collecting spillage .
Orientations Futures
Propriétés
IUPAC Name |
(1,2-dibromo-2,2-dichloroethyl) bis(trideuteriomethyl) phosphate |
Source
|
---|---|---|
Source | PubChem | |
URL | https://pubchem.ncbi.nlm.nih.gov | |
Description | Data deposited in or computed by PubChem | |
InChI |
InChI=1S/C4H7Br2Cl2O4P/c1-10-13(9,11-2)12-3(5)4(6,7)8/h3H,1-2H3/i1D3,2D3 |
Source
|
Source | PubChem | |
URL | https://pubchem.ncbi.nlm.nih.gov | |
Description | Data deposited in or computed by PubChem | |
InChI Key |
BUYMVQAILCEWRR-WFGJKAKNSA-N |
Source
|
Source | PubChem | |
URL | https://pubchem.ncbi.nlm.nih.gov | |
Description | Data deposited in or computed by PubChem | |
Canonical SMILES |
COP(=O)(OC)OC(C(Cl)(Cl)Br)Br |
Source
|
Source | PubChem | |
URL | https://pubchem.ncbi.nlm.nih.gov | |
Description | Data deposited in or computed by PubChem | |
Isomeric SMILES |
[2H]C([2H])([2H])OP(=O)(OC(C(Cl)(Cl)Br)Br)OC([2H])([2H])[2H] |
Source
|
Source | PubChem | |
URL | https://pubchem.ncbi.nlm.nih.gov | |
Description | Data deposited in or computed by PubChem | |
Molecular Formula |
C4H7Br2Cl2O4P |
Source
|
Source | PubChem | |
URL | https://pubchem.ncbi.nlm.nih.gov | |
Description | Data deposited in or computed by PubChem | |
DSSTOX Substance ID |
DTXSID10676053 |
Source
|
Record name | 1,2-Dibromo-2,2-dichloroethyl bis[(~2~H_3_)methyl] phosphate | |
Source | EPA DSSTox | |
URL | https://comptox.epa.gov/dashboard/DTXSID10676053 | |
Description | DSSTox provides a high quality public chemistry resource for supporting improved predictive toxicology. | |
Molecular Weight |
386.82 g/mol |
Source
|
Source | PubChem | |
URL | https://pubchem.ncbi.nlm.nih.gov | |
Description | Data deposited in or computed by PubChem | |
CAS No. |
1216605-57-0 |
Source
|
Record name | 1,2-Dibromo-2,2-dichloroethyl bis[(~2~H_3_)methyl] phosphate | |
Source | EPA DSSTox | |
URL | https://comptox.epa.gov/dashboard/DTXSID10676053 | |
Description | DSSTox provides a high quality public chemistry resource for supporting improved predictive toxicology. | |
Avertissement et informations sur les produits de recherche in vitro
Veuillez noter que tous les articles et informations sur les produits présentés sur BenchChem sont destinés uniquement à des fins informatives. Les produits disponibles à l'achat sur BenchChem sont spécifiquement conçus pour des études in vitro, qui sont réalisées en dehors des organismes vivants. Les études in vitro, dérivées du terme latin "in verre", impliquent des expériences réalisées dans des environnements de laboratoire contrôlés à l'aide de cellules ou de tissus. Il est important de noter que ces produits ne sont pas classés comme médicaments et n'ont pas reçu l'approbation de la FDA pour la prévention, le traitement ou la guérison de toute condition médicale, affection ou maladie. Nous devons souligner que toute forme d'introduction corporelle de ces produits chez les humains ou les animaux est strictement interdite par la loi. Il est essentiel de respecter ces directives pour assurer la conformité aux normes légales et éthiques en matière de recherche et d'expérimentation.