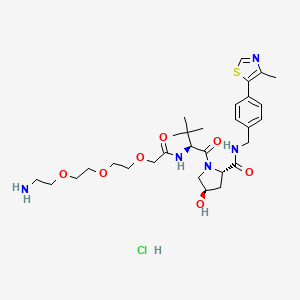
E3 ligase Ligand-Linker Conjugates 5
Vue d'ensemble
Description
VH032-PEG3-NH2 (chlorhydrate) est un conjugué ligand-lieur de ligase E3 synthétisé qui intègre le ligand von Hippel-Lindau (VHL) basé sur (S,R,S)-AHPC et un lieur de polyéthylène glycol (PEG) à 3 unités. Ce composé est principalement utilisé dans la technologie des chimères de ciblage de la protéolyse (PROTAC), qui est une approche nouvelle dans la découverte et le développement de médicaments .
Mécanisme D'action
Target of Action
The primary target of E3 ligase Ligand-Linker Conjugates 5 is the Cereblon (CRBN) protein . CRBN is a substrate receptor of the E3 ubiquitin ligase complex . E3 ubiquitin ligases are a large family of enzymes that play an essential role in catalyzing the ubiquitination process and transferring ubiquitin protein to attach the lysine site of targeted substrates .
Mode of Action
This compound serves as a Cereblon ligand to recruit CRBN protein . It is a conjugate of E3 ligase ligand and linker, consisting of Thalidomide and the corresponding linker . The compound can selectively attach ubiquitin to lysine, serine, threonine, or cysteine residues to the specific substrates .
Biochemical Pathways
The compound is involved in the ubiquitin-proteasome system (UPS) . The UPS is a cascade reaction and an important way for short-lived, misfolded, and damaged proteins degradation . As reported, the UPS can regulate degradation of over 80% proteins in cells and its dysregulation has been revealed in most hallmarks of cancer .
Pharmacokinetics
The compound is a key intermediate for the synthesis of complete protac molecules , which are known for their ability to degrade target proteins within cells. The ADME properties of the compound and their impact on bioavailability would need further investigation.
Result of Action
The result of the compound’s action is the degradation of target proteins within cells. This is achieved by the ubiquitination of the target proteins, which marks them for degradation by the proteasome . This process is crucial in regulating various biological processes and cellular responses to stress signals associated with cancer development .
Action Environment
The action of this compound can be influenced by various environmental factors. For instance, the expression levels of E3 ligases and their substrates can vary depending on the cellular environment Additionally, the stability and efficacy of the compound could be affected by factors such as pH, temperature, and the presence of other interacting molecules
Méthodes De Préparation
Voies de synthèse et conditions de réaction
La synthèse de VH032-PEG3-NH2 (chlorhydrate) implique plusieurs étapes, en commençant par la préparation du ligand VHL basé sur (S,R,S)-AHPC. Ce ligand est ensuite conjugué avec un lieur PEG à 3 unités par une série de réactions chimiques, notamment la formation de liaison amide et les étapes de purification .
Méthodes de production industrielle
La production industrielle de VH032-PEG3-NH2 (chlorhydrate) suit des voies de synthèse similaires, mais à plus grande échelle. Le processus implique l'optimisation des conditions de réaction pour garantir un rendement et une pureté élevés. Cela comprend le contrôle de la température, du pH et du temps de réaction, ainsi que l'utilisation de techniques de purification avancées telles que la chromatographie .
Analyse Des Réactions Chimiques
Types de réactions
VH032-PEG3-NH2 (chlorhydrate) subit diverses réactions chimiques, notamment :
Oxydation : Le composé peut être oxydé dans des conditions spécifiques pour former des dérivés oxydés.
Réduction : Des réactions de réduction peuvent être effectuées pour modifier les groupes fonctionnels présents dans le composé.
Substitution : Le composé peut subir des réactions de substitution nucléophile, où les groupes fonctionnels sont remplacés par d'autres nucléophiles.
Réactifs et conditions courants
Les réactifs courants utilisés dans ces réactions comprennent des agents oxydants comme le peroxyde d'hydrogène, des agents réducteurs comme le borohydrure de sodium et des nucléophiles comme les amines et les thiols. Les conditions de réaction varient en fonction de la transformation souhaitée, mais impliquent généralement des températures contrôlées et des atmosphères inertes .
Principaux produits formés
Les principaux produits formés à partir de ces réactions dépendent des réactifs et des conditions spécifiques utilisés. Par exemple, l'oxydation peut donner des dérivés hydroxylés, tandis que les réactions de substitution peuvent produire divers analogues substitués .
Applications de la recherche scientifique
VH032-PEG3-NH2 (chlorhydrate) a une large gamme d'applications de recherche scientifique :
Chimie : Utilisé comme élément constitutif dans la synthèse de molécules complexes et comme réactif dans diverses réactions chimiques.
Biologie : Utilisé dans la technologie PROTAC pour cibler et dégrader des protéines spécifiques, aidant à l'étude de la fonction et de la régulation des protéines.
Médecine : Investigué pour son potentiel dans la découverte de médicaments, en particulier dans le développement de traitements pour des maladies telles que le cancer en ciblant et en dégradant les protéines responsables de la maladie.
Industrie : Utilisé dans le développement de nouveaux matériaux et procédés chimiques
Mécanisme d'action
VH032-PEG3-NH2 (chlorhydrate) exerce ses effets par le biais du mécanisme PROTAC. Il recrute la ligase E3 ubiquitine VHL sur la protéine cible, ce qui conduit à l'ubiquitination et à la dégradation subséquente de la protéine cible par le protéasome. Ce processus implique la formation d'un complexe ternaire entre le PROTAC, la protéine cible et la ligase E3, ce qui entraîne la dégradation sélective de la protéine cible .
Applications De Recherche Scientifique
VH032-PEG3-NH2 (hydrochloride) has a wide range of scientific research applications:
Chemistry: Used as a building block in the synthesis of complex molecules and as a reagent in various chemical reactions.
Biology: Employed in PROTAC technology to target and degrade specific proteins, aiding in the study of protein function and regulation.
Medicine: Investigated for its potential in drug discovery, particularly in developing treatments for diseases such as cancer by targeting and degrading disease-causing proteins.
Industry: Utilized in the development of new materials and chemical processes
Comparaison Avec Des Composés Similaires
Composés similaires
VH032 : Un inhibiteur de la ligase E3 ubiquitine VHL, utilisé dans des applications similaires à celles de VH032-PEG3-NH2 (chlorhydrate).
AHPC-PEG3-NH2 : Un autre conjugué ligand-lieur de ligase E3 utilisé dans la technologie PROTAC
Unicité
VH032-PEG3-NH2 (chlorhydrate) est unique en raison de sa combinaison spécifique du ligand VHL basé sur (S,R,S)-AHPC et du lieur PEG à 3 unités. Cette structure permet un recrutement efficace de la ligase E3 VHL et une dégradation efficace des protéines cibles, ce qui en fait un outil précieux dans la technologie PROTAC et la découverte de médicaments .
Propriétés
IUPAC Name |
(2S,4R)-1-[(2S)-2-[[2-[2-[2-(2-aminoethoxy)ethoxy]ethoxy]acetyl]amino]-3,3-dimethylbutanoyl]-4-hydroxy-N-[[4-(4-methyl-1,3-thiazol-5-yl)phenyl]methyl]pyrrolidine-2-carboxamide;hydrochloride | |
---|---|---|
Source | PubChem | |
URL | https://pubchem.ncbi.nlm.nih.gov | |
Description | Data deposited in or computed by PubChem | |
InChI |
InChI=1S/C30H45N5O7S.ClH/c1-20-26(43-19-33-20)22-7-5-21(6-8-22)16-32-28(38)24-15-23(36)17-35(24)29(39)27(30(2,3)4)34-25(37)18-42-14-13-41-12-11-40-10-9-31;/h5-8,19,23-24,27,36H,9-18,31H2,1-4H3,(H,32,38)(H,34,37);1H/t23-,24+,27-;/m1./s1 | |
Source | PubChem | |
URL | https://pubchem.ncbi.nlm.nih.gov | |
Description | Data deposited in or computed by PubChem | |
InChI Key |
ZOYHUTRHKHRRPK-QVRKWNSCSA-N | |
Source | PubChem | |
URL | https://pubchem.ncbi.nlm.nih.gov | |
Description | Data deposited in or computed by PubChem | |
Canonical SMILES |
CC1=C(SC=N1)C2=CC=C(C=C2)CNC(=O)C3CC(CN3C(=O)C(C(C)(C)C)NC(=O)COCCOCCOCCN)O.Cl | |
Source | PubChem | |
URL | https://pubchem.ncbi.nlm.nih.gov | |
Description | Data deposited in or computed by PubChem | |
Isomeric SMILES |
CC1=C(SC=N1)C2=CC=C(C=C2)CNC(=O)[C@@H]3C[C@H](CN3C(=O)[C@H](C(C)(C)C)NC(=O)COCCOCCOCCN)O.Cl | |
Source | PubChem | |
URL | https://pubchem.ncbi.nlm.nih.gov | |
Description | Data deposited in or computed by PubChem | |
Molecular Formula |
C30H46ClN5O7S | |
Source | PubChem | |
URL | https://pubchem.ncbi.nlm.nih.gov | |
Description | Data deposited in or computed by PubChem | |
Molecular Weight |
656.2 g/mol | |
Source | PubChem | |
URL | https://pubchem.ncbi.nlm.nih.gov | |
Description | Data deposited in or computed by PubChem | |
Retrosynthesis Analysis
AI-Powered Synthesis Planning: Our tool employs the Template_relevance Pistachio, Template_relevance Bkms_metabolic, Template_relevance Pistachio_ringbreaker, Template_relevance Reaxys, Template_relevance Reaxys_biocatalysis model, leveraging a vast database of chemical reactions to predict feasible synthetic routes.
One-Step Synthesis Focus: Specifically designed for one-step synthesis, it provides concise and direct routes for your target compounds, streamlining the synthesis process.
Accurate Predictions: Utilizing the extensive PISTACHIO, BKMS_METABOLIC, PISTACHIO_RINGBREAKER, REAXYS, REAXYS_BIOCATALYSIS database, our tool offers high-accuracy predictions, reflecting the latest in chemical research and data.
Strategy Settings
Precursor scoring | Relevance Heuristic |
---|---|
Min. plausibility | 0.01 |
Model | Template_relevance |
Template Set | Pistachio/Bkms_metabolic/Pistachio_ringbreaker/Reaxys/Reaxys_biocatalysis |
Top-N result to add to graph | 6 |
Feasible Synthetic Routes
Avertissement et informations sur les produits de recherche in vitro
Veuillez noter que tous les articles et informations sur les produits présentés sur BenchChem sont destinés uniquement à des fins informatives. Les produits disponibles à l'achat sur BenchChem sont spécifiquement conçus pour des études in vitro, qui sont réalisées en dehors des organismes vivants. Les études in vitro, dérivées du terme latin "in verre", impliquent des expériences réalisées dans des environnements de laboratoire contrôlés à l'aide de cellules ou de tissus. Il est important de noter que ces produits ne sont pas classés comme médicaments et n'ont pas reçu l'approbation de la FDA pour la prévention, le traitement ou la guérison de toute condition médicale, affection ou maladie. Nous devons souligner que toute forme d'introduction corporelle de ces produits chez les humains ou les animaux est strictement interdite par la loi. Il est essentiel de respecter ces directives pour assurer la conformité aux normes légales et éthiques en matière de recherche et d'expérimentation.