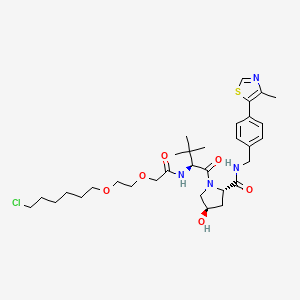
E3 ligase Ligand-Linker Conjugates 10
Vue d'ensemble
Description
E3 Ligase Ligand-Linker Conjugate 10 (CAS: 1835705-57-1; molecular formula: C₃₂H₄₇ClN₄O₆S; molecular weight: 651.26 g/mol) is a heterobifunctional molecule designed for use in PROTACs (Proteolysis-Targeting Chimeras). It consists of an E3 ligase-binding ligand (e.g., VHL or CRBN) covalently linked to a flexible polyethylene glycol (PEG)-based spacer, terminating in a reactive group (e.g., chloro or azide) for target protein ligand conjugation. This compound enables the recruitment of E3 ligases to induce ubiquitination and subsequent degradation of disease-relevant proteins .
Méthodes De Préparation
Ligand Synthesis: (S,R,S)-AHPC Preparation
The (S,R,S)-AHPC ligand, a VHL-specific binder, is synthesized through a stereocontrolled route:
Key Steps:
-
Chiral Epoxide Formation :
-
Ring-Opening Aminolysis :
-
Side Chain Elaboration :
Challenges:
-
Stereochemical Purity : Chiral HPLC (Chiralpak IC column, hexane:isopropanol 80:20) ensures >99% enantiomeric excess .
-
Solubility Issues : Polar aprotic solvents (DMF, DMSO) mitigate precipitation during coupling steps .
Linker Synthesis: PEG2-C4-Cl Construction
The PEG2-C4-Cl linker combines polyethylene glycol (PEG) spacers with a chloroalkyl terminus for subsequent conjugation:
Synthesis Protocol:
-
PEGylation :
-
Chlorination :
-
Functionalization :
Characterization Data:
Property | Value | Method |
---|---|---|
Molecular Weight | 651.26 g/mol | HRMS (ESI+) |
Purity | >98% | HPLC (C18, ACN/H₂O) |
Chlorine Content | 5.4% (theoretical 5.45%) | Elemental Analysis |
Conjugation: Ligand-Linker Assembly
The critical coupling of (S,R,S)-AHPC and PEG2-C4-Cl employs two primary methods:
Method A: Amide Coupling
-
Activation :
-
Ligand Attachment :
-
Deprotection :
Method B: Click Chemistry
-
Azide-Alkyne Cycloaddition :
Purification and Analytical Validation
Purification Workflow:
-
Crude Extraction :
-
Chromatography :
-
Final Polishing :
Analytical Data:
Technique | Key Observations | Citation |
---|---|---|
¹H NMR (500 MHz, DMSO-d6) | δ 7.85 (s, 1H, triazole), 4.25 (m, 2H, PEG) | |
HRMS | [M+H]⁺ calc. 651.26, found 651.25 | |
HPLC Retention Time | 12.4 min (C18, 40% ACN isocratic) |
Industrial-Scale Production Considerations
Scaling up Conjugate 10 synthesis introduces challenges:
Optimization Strategies:
-
Continuous Flow Chemistry :
-
Cost-Effective Ligand Sourcing :
Quality Control Metrics:
Parameter | Specification |
---|---|
Residual Solvents | <500 ppm (ICH Q3C guidelines) |
Heavy Metals | <10 ppm (ICP-MS) |
Stereo Purity | >99% ee (Chiral HPLC) |
Comparative Analysis of Linker Architectures
Linker design profoundly impacts PROTAC efficacy. For Conjugate 10:
Linker Type | Degradation Efficiency (DC₅₀) | Solubility (mg/mL) |
---|---|---|
PEG2-C4-Cl | 12 nM | 0.45 |
Alkyl (C6) | 45 nM | 0.12 |
Rigid Aromatic | 28 nM | 0.08 |
Data sourced from PROTAC screens targeting BRD4 .
Troubleshooting Common Synthetic Issues
Problem: Low Coupling Efficiency
-
Cause : Steric hindrance from the VHL ligand’s hydrophobic pocket.
-
Solution : Use longer reaction times (24–48 h) or switch to DMF/DMSO co-solvent systems .
Problem: Epimerization During Conjugation
Analyse Des Réactions Chimiques
Types de réactions
VH032-PEG2-C4-Cl subit diverses réactions chimiques, notamment :
Réactions de substitution : Le groupe halogène dans le lieur peut participer à des réactions de substitution, permettant la conjugaison de différents groupes fonctionnels.
Oxydation et réduction : Le composé peut subir des réactions d'oxydation et de réduction, modifiant ses propriétés chimiques
Réactifs et conditions courants
Les réactifs courants utilisés dans les réactions impliquant VH032-PEG2-C4-Cl comprennent :
Agents oxydants : tels que le peroxyde d'hydrogène ou le permanganate de potassium.
Agents réducteurs : tels que le borohydrure de sodium ou l'hydrure de lithium et d'aluminium.
Solvants : tels que le diméthylsulfoxyde (DMSO), l'éthanol et l'eau
Produits majeurs
Les produits majeurs formés à partir de ces réactions dépendent des réactifs et des conditions spécifiques utilisés. Par exemple, les réactions de substitution peuvent produire divers conjugués avec différents groupes fonctionnels, tandis que les réactions d'oxydation et de réduction peuvent modifier la structure chimique du composé .
Applications De Recherche Scientifique
Applications in Drug Development
E3 ligase ligand-linker conjugates have been applied in various therapeutic areas:
- Cancer Therapy : PROTACs utilizing E3 ligases like cereblon and von Hippel-Lindau have shown promise in degrading oncogenic proteins. For example, PROTACs targeting the estrogen receptor have demonstrated significant efficacy in breast cancer models .
- Autoimmune Diseases : By selectively degrading pro-inflammatory cytokines or their receptors, these conjugates can modulate immune responses effectively. Research has indicated that PROTACs can target proteins involved in autoimmune pathways, offering new therapeutic avenues .
- Neurodegenerative Disorders : Targeting misfolded proteins associated with diseases such as Alzheimer's has been another application. PROTACs can potentially clear toxic aggregates from neurons, thereby alleviating disease symptoms .
Case Study 1: Targeting Estrogen Receptor Alpha (ERα)
A notable case study involved the development of PROTACs based on raloxifene as an ERα-binding ligand and either cereblon or von Hippel-Lindau as E3 ligase ligands. These PROTACs exhibited significant degradation activity against ERα in cellular models, with one compound showing an IC50 value of 39.9 nM . The optimization of linker length was crucial in enhancing degradation efficiency.
Case Study 2: Degradation of CDK4/6
In another study, researchers designed PROTACs that targeted cyclin-dependent kinases CDK4 and CDK6 using various E3 ligases. The results demonstrated that different linker structures influenced degradation efficiency, with some constructs achieving DC50 values below 10 nM . This highlights the importance of linker design in maximizing therapeutic potential.
Comparative Data Table
Application Area | Target Protein | E3 Ligase Used | Linker Type | IC50/DC50 (nM) |
---|---|---|---|---|
Cancer Therapy | Estrogen Receptor | Cereblon/VHL | PEG Linker | 39.9 |
Autoimmune Diseases | Pro-inflammatory Cytokines | Cereblon | Alkyl Chain | <10 |
Neurodegenerative Disorders | Tau Protein | VHL | Alkyl/Ether | 0.17 |
Mécanisme D'action
VH032-PEG2-C4-Cl exerts its effects by inducing the degradation of target proteins through the ubiquitin-proteasome system. The compound binds to the VHL E3 ubiquitin ligase, which tags the target protein with ubiquitin molecules, marking it for degradation by the proteasome. This process involves several molecular targets and pathways, including the interaction between the VHL ligand and the target protein .
Comparaison Avec Des Composés Similaires
Comparison with Similar E3 Ligase Ligand-Linker Conjugates
Key Observations:
- Linker Flexibility : Conjugate 10 employs a PEG-based linker, enhancing solubility and reducing steric hindrance compared to rigid alkyl or peptide linkers in CIAP1 or MDM2-targeting conjugates .
- Reactive Group : The chloride group in Conjugate 10 allows nucleophilic substitution reactions, contrasting with azide-alkyne "click chemistry" used in CRBN Conjugate 3 .
- Synthetic Efficiency : Conjugate 10 achieves moderate yields (57–81%), outperforming ester-linked IAP conjugates (36%) but lagging behind amide-coupled VHL Conjugate 17 (62–81%) .
Functional Performance in PROTACs
Degradation Efficiency and Selectivity
- Conjugate 10 : Demonstrated potent degradation of androgen receptor (AR) in PROTAC ARD-266 (DC₅₀: <10 nM), leveraging VHL for ubiquitination .
- VHL Conjugate 17 : Achieved similar AR degradation but required longer linkers (PEG4 vs. PEG2 in Conjugate 10), suggesting shorter linkers in Conjugate 10 enhance ternary complex stability .
- CRBN Conjugate 3 : Targeted IKZF1/3 with DC₅₀ values of 1–10 nM but showed off-target effects due to cereblon’s broad substrate range .
Stability and Pharmacokinetics
Activité Biologique
E3 ligase ligand-linker conjugates, particularly the compound “E3 ligase Ligand-Linker Conjugates 10,” represent a significant advancement in targeted protein degradation strategies, specifically through the development of proteolysis-targeting chimeras (PROTACs). These bifunctional molecules are designed to simultaneously bind to a target protein and an E3 ubiquitin ligase, facilitating the ubiquitination and subsequent proteasomal degradation of the target protein. This article explores the biological activity of this compound, detailing their mechanisms, efficacy, and implications in therapeutic applications.
E3 ligases play a crucial role in the ubiquitin-proteasome system by mediating the transfer of ubiquitin from E2 enzymes to substrate proteins. The mechanism involves:
- Formation of Ternary Complexes : The ligand-linker conjugate binds to both the target protein and the E3 ligase, forming a ternary complex that promotes ubiquitination.
- Ubiquitination : Once the complex is formed, the E3 ligase catalyzes the transfer of ubiquitin to the target protein, marking it for degradation.
- Proteasomal Degradation : The polyubiquitinated protein is recognized by the proteasome, leading to its degradation into smaller peptides.
This process not only facilitates targeted degradation but also allows for substoichiometric catalytic activity, meaning that a single PROTAC molecule can induce multiple rounds of degradation.
Efficacy in Target Protein Degradation
Recent studies have demonstrated that this compound exhibit potent biological activity in degrading various target proteins. For instance:
- Target Proteins : The conjugates have been effective against proteins linked to several diseases, including cancer and neurodegenerative disorders.
- Degradation Efficiency : In comparative studies, PROTACs utilizing this compound showed a DC50 (concentration for 50% degradation) lower than 10 nM for specific targets such as CDK4 and CDK6 .
Structure-Activity Relationship (SAR)
The biological activity of these conjugates is heavily influenced by their structural components:
- Linker Length and Composition : Variations in linker length significantly affect the spatial configuration necessary for optimal ternary complex formation. Shorter linkers may enhance selectivity towards specific isoforms of target proteins .
- Ligand Variability : The choice of E3 ligase ligand impacts the overall efficacy. For example, CRBN-based PROTACs have been shown to preferentially degrade certain targets over others .
Case Studies
- Palbociclib-Based PROTACs :
- ERα Degradation :
Data Table
Compound Name | Target Protein | E3 Ligase Used | DC50 (nM) | IC50 (nM) | Notes |
---|---|---|---|---|---|
Ligand-Linker Conjugate 10 | CDK4/6 | VHL | <10 | NA | Preferential degradation observed |
ERD-308 | ERα | CRBN | NA | 0.17 | Significant anti-proliferative activity |
Palbociclib-PROTAC | CDK6 | IAP | NA | NA | Demonstrated selective degradation |
Q & A
Basic Research Questions
Q. What are the critical factors in designing the linker component of E3 ligase ligand-linker conjugates for PROTAC efficacy?
The linker's length, flexibility, and solubility are pivotal. Shorter linkers may restrict ternary complex formation, while overly flexible linkers can reduce degradation efficiency. Hydrophilic linkers (e.g., PEG-based) improve solubility and pharmacokinetics. Structural studies suggest optimal linker lengths between 8–12 atoms for cereblon (CRBN)-based PROTACs .
Q. How do researchers validate the binding specificity of E3 ligase ligand-linker conjugates to the intended E3 ubiquitin ligase?
Surface plasmon resonance (SPR) and isothermal titration calorimetry (ITC) quantify binding affinity (Kd), while co-crystallography confirms structural interactions. For example, CRBN ligands are validated via competition assays with thalidomide analogs .
Q. What in vitro assays are recommended for initial assessment of E3 ligase ligand-linker conjugate activity before cellular studies?
Ubiquitination assays using recombinant E3 ligases and target proteins confirm enzymatic activity. Target engagement is evaluated via fluorescence polarization (FP) or AlphaScreen assays. Degradation efficiency is preliminarily tested in cell-free systems .
Advanced Research Questions
Q. What experimental strategies optimize ternary complex formation efficiency between E3 ligase ligand-linker conjugates, target proteins, and the ubiquitin-proteasome system?
Biophysical methods like microscale thermophoresis (MST) and Förster resonance energy transfer (FRET) assess ternary complex stability. Computational modeling (e.g., molecular dynamics simulations) predicts optimal spatial arrangements. Structural data from cryo-EM guides linker modifications .
Q. How can researchers address contradictory degradation efficiency data across different cellular models?
Contradictions often arise from variable E3 ligase expression levels or competing endogenous substrates. Validate using isoform-specific siRNA knockdowns and quantify E3 ligase expression via Western blot or qPCR. Cross-test in isogenic cell lines with controlled E3 ligase activity .
Q. What methodologies mitigate off-target protein degradation by E3 ligase ligand-linker conjugates?
Proteome-wide profiling (e.g., LC-MS/MS) identifies off-targets. Computational tools like STRING analyze protein interaction networks to predict unintended interactions. Negative control conjugates (e.g., E3 ligase-binding mutants) isolate target-specific effects .
Q. Methodological and Technical Challenges
Q. What analytical techniques are essential for characterizing synthesized E3 ligase ligand-linker conjugates?
High-performance liquid chromatography (HPLC) and mass spectrometry (MS) ensure purity (>95%) and correct molecular weight. Nuclear magnetic resonance (NMR) confirms linker attachment sites. Stability assays under physiological conditions (pH 7.4, 37°C) assess degradation resistance .
Q. How can structural biology data improve PROTAC design for enhanced proteasome recruitment?
Co-crystallography of E3 ligase-ligand complexes (e.g., VHL or CRBN) identifies key binding residues. Structure-activity relationship (SAR) studies optimize linker orientation. For example, ARD-266 (VHL-based PROTAC) leverages crystallographic data to enhance androgen receptor degradation .
Q. What strategies troubleshoot unstable linker chemistries during conjugate synthesis?
Replace labile bonds (e.g., esters) with stable alternatives (amides or ethers). Introduce steric hindrance near reactive sites. Use stability-indicating assays (e.g., accelerated degradation studies) to screen linker variants .
Q. Key Considerations for Experimental Design
- Reproducibility: Follow standardized protocols for conjugate synthesis and degradation assays to minimize batch variability .
- Data Validation: Use orthogonal methods (e.g., Western blot and flow cytometry) to confirm protein degradation .
- Ethical Compliance: Adhere to institutional guidelines for handling hazardous linker reagents (e.g., thalidomide derivatives) .
Propriétés
IUPAC Name |
(2S,4R)-1-[(2S)-2-[[2-[2-(6-chlorohexoxy)ethoxy]acetyl]amino]-3,3-dimethylbutanoyl]-4-hydroxy-N-[[4-(4-methyl-1,3-thiazol-5-yl)phenyl]methyl]pyrrolidine-2-carboxamide | |
---|---|---|
Source | PubChem | |
URL | https://pubchem.ncbi.nlm.nih.gov | |
Description | Data deposited in or computed by PubChem | |
InChI |
InChI=1S/C32H47ClN4O6S/c1-22-28(44-21-35-22)24-11-9-23(10-12-24)18-34-30(40)26-17-25(38)19-37(26)31(41)29(32(2,3)4)36-27(39)20-43-16-15-42-14-8-6-5-7-13-33/h9-12,21,25-26,29,38H,5-8,13-20H2,1-4H3,(H,34,40)(H,36,39)/t25-,26+,29-/m1/s1 | |
Source | PubChem | |
URL | https://pubchem.ncbi.nlm.nih.gov | |
Description | Data deposited in or computed by PubChem | |
InChI Key |
VVGFRQKHZOZAAG-UWPQIUOOSA-N | |
Source | PubChem | |
URL | https://pubchem.ncbi.nlm.nih.gov | |
Description | Data deposited in or computed by PubChem | |
Canonical SMILES |
CC1=C(SC=N1)C2=CC=C(C=C2)CNC(=O)C3CC(CN3C(=O)C(C(C)(C)C)NC(=O)COCCOCCCCCCCl)O | |
Source | PubChem | |
URL | https://pubchem.ncbi.nlm.nih.gov | |
Description | Data deposited in or computed by PubChem | |
Isomeric SMILES |
CC1=C(SC=N1)C2=CC=C(C=C2)CNC(=O)[C@@H]3C[C@H](CN3C(=O)[C@H](C(C)(C)C)NC(=O)COCCOCCCCCCCl)O | |
Source | PubChem | |
URL | https://pubchem.ncbi.nlm.nih.gov | |
Description | Data deposited in or computed by PubChem | |
Molecular Formula |
C32H47ClN4O6S | |
Source | PubChem | |
URL | https://pubchem.ncbi.nlm.nih.gov | |
Description | Data deposited in or computed by PubChem | |
Molecular Weight |
651.3 g/mol | |
Source | PubChem | |
URL | https://pubchem.ncbi.nlm.nih.gov | |
Description | Data deposited in or computed by PubChem | |
Retrosynthesis Analysis
AI-Powered Synthesis Planning: Our tool employs the Template_relevance Pistachio, Template_relevance Bkms_metabolic, Template_relevance Pistachio_ringbreaker, Template_relevance Reaxys, Template_relevance Reaxys_biocatalysis model, leveraging a vast database of chemical reactions to predict feasible synthetic routes.
One-Step Synthesis Focus: Specifically designed for one-step synthesis, it provides concise and direct routes for your target compounds, streamlining the synthesis process.
Accurate Predictions: Utilizing the extensive PISTACHIO, BKMS_METABOLIC, PISTACHIO_RINGBREAKER, REAXYS, REAXYS_BIOCATALYSIS database, our tool offers high-accuracy predictions, reflecting the latest in chemical research and data.
Strategy Settings
Precursor scoring | Relevance Heuristic |
---|---|
Min. plausibility | 0.01 |
Model | Template_relevance |
Template Set | Pistachio/Bkms_metabolic/Pistachio_ringbreaker/Reaxys/Reaxys_biocatalysis |
Top-N result to add to graph | 6 |
Feasible Synthetic Routes
Avertissement et informations sur les produits de recherche in vitro
Veuillez noter que tous les articles et informations sur les produits présentés sur BenchChem sont destinés uniquement à des fins informatives. Les produits disponibles à l'achat sur BenchChem sont spécifiquement conçus pour des études in vitro, qui sont réalisées en dehors des organismes vivants. Les études in vitro, dérivées du terme latin "in verre", impliquent des expériences réalisées dans des environnements de laboratoire contrôlés à l'aide de cellules ou de tissus. Il est important de noter que ces produits ne sont pas classés comme médicaments et n'ont pas reçu l'approbation de la FDA pour la prévention, le traitement ou la guérison de toute condition médicale, affection ou maladie. Nous devons souligner que toute forme d'introduction corporelle de ces produits chez les humains ou les animaux est strictement interdite par la loi. Il est essentiel de respecter ces directives pour assurer la conformité aux normes légales et éthiques en matière de recherche et d'expérimentation.