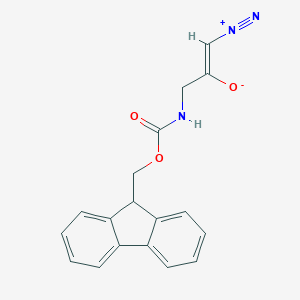
Fmoc-Gly-CHN2
Vue d'ensemble
Description
Fmoc-Gly-CHN2 (CAS: 275816-73-4) is an Fmoc (9-fluorenylmethoxycarbonyl)-protected glycine derivative with the molecular formula C₁₈H₁₅N₃O₃ and a molecular weight of 321.33 g/mol . Glycine, being the smallest and least sterically hindered amino acid, confers high flexibility when incorporated into peptides. This compound is primarily utilized in solid-phase peptide synthesis (SPPS) and protein engineering, where its minimal steric bulk facilitates efficient coupling reactions . This compound also serves as an unconventional amino acid analog, enabling structural and functional studies of proteins . Its diazomethylene (CHN₂) group may act as a reactive site for further chemical modifications, though this requires validation through spectral techniques like NMR or mass spectrometry .
Méthodes De Préparation
Synthetic Routes and Reaction Conditions
The preparation of Fmoc-Gly-CHN2 involves the protection of the glycine amino group with the fluorenylmethyloxycarbonyl (Fmoc) group. This can be achieved by reacting glycine with fluorenylmethyloxycarbonyl chloride (Fmoc-Cl) in the presence of a base such as sodium bicarbonate in an aqueous dioxane solution . The diazomethane group is then introduced to the protected glycine to form this compound.
Industrial Production Methods
Industrial production of this compound follows similar synthetic routes but on a larger scale. The process involves the use of automated solid-phase peptide synthesis (SPPS) equipment to ensure high yield and purity. The reaction conditions are optimized to minimize side reactions and maximize the efficiency of the synthesis .
Analyse Des Réactions Chimiques
Types of Reactions
Fmoc-Gly-CHN2 undergoes several types of chemical reactions, including:
Substitution Reactions: The diazomethane group can participate in nucleophilic substitution reactions.
Deprotection Reactions: The Fmoc group can be removed under basic conditions, typically using piperidine in N,N-dimethylformamide (DMF).
Common Reagents and Conditions
Substitution Reactions: Common reagents include nucleophiles such as amines or alcohols.
Deprotection Reactions: Piperidine in DMF is commonly used to remove the Fmoc group.
Major Products Formed
Substitution Reactions: The major products depend on the nucleophile used. For example, reacting with an amine would yield an amide derivative.
Deprotection Reactions: The removal of the Fmoc group yields free glycine derivatives.
Applications De Recherche Scientifique
Peptide Synthesis
Fmoc-Gly-CHN2 is primarily utilized in solid-phase peptide synthesis (SPPS). The Fmoc group serves as a temporary protecting group for the amino group of glycine, allowing for the sequential addition of amino acids to form peptides. This method is advantageous for several reasons:
- Efficiency : The use of Fmoc chemistry allows for rapid deprotection and coupling reactions, significantly speeding up the synthesis process.
- Purity : SPPS using this compound often results in high-purity peptides due to the controlled environment of solid-phase synthesis.
- Diversity : Researchers can easily introduce various amino acids to create diverse peptide sequences tailored for specific biological functions.
Drug Development
In drug development, this compound is employed to create peptide-based therapeutics. Peptides have shown promise in treating various diseases due to their specificity and lower toxicity compared to traditional small-molecule drugs. Key applications include:
- Targeted Therapies : Peptides synthesized with this compound can be designed to target specific receptors or pathways involved in disease processes, enhancing therapeutic efficacy.
- Vaccine Development : this compound-derived peptides are used in vaccine formulations, where they can serve as epitopes that stimulate immune responses against pathogens or cancer cells.
- Bioconjugation : The reactive nature of the azide group in this compound allows for bioconjugation with other biomolecules (e.g., antibodies or nanoparticles), facilitating targeted delivery systems.
Biochemical Research
The compound is also pivotal in biochemical research, particularly in studying protein interactions and dynamics. Its applications include:
- Protein Labeling : this compound can be incorporated into proteins as a label for tracking interactions or conformational changes using techniques like fluorescence or mass spectrometry.
- Enzyme Substrate Studies : Researchers utilize peptides derived from this compound to investigate enzyme specificity and kinetics, providing insights into metabolic pathways.
- Structural Biology : The compound's incorporation into peptides aids in X-ray crystallography and NMR studies, helping elucidate protein structures and interactions.
Case Study 1: Peptide Therapeutics
A study demonstrated the successful synthesis of a peptide inhibitor targeting a specific enzyme involved in cancer progression using this compound. The synthesized peptide exhibited high binding affinity and specificity, highlighting the potential of Fmoc-based methodologies in developing targeted cancer therapies.
Case Study 2: Vaccine Development
In another case, researchers used this compound-derived peptides as vaccine candidates against viral infections. The peptides were shown to elicit robust immune responses in animal models, paving the way for further clinical trials.
Mécanisme D'action
The mechanism of action of Fmoc-Gly-CHN2 involves the protection of the amino group of glycine with the Fmoc group, which prevents unwanted side reactions during peptide synthesis. The diazomethane group allows for further functionalization of the glycine derivative. The Fmoc group can be removed under basic conditions, allowing for the incorporation of glycine into peptides .
Comparaison Avec Des Composés Similaires
Structural and Functional Differences
The following table summarizes key structural and application differences between Fmoc-Gly-CHN2 and related Fmoc-protected amino acids or linkers:
Key Research Findings
Reactivity and Stability
- This compound lacks halogen or fluorine substituents, limiting its utility in selective cross-coupling reactions compared to 3-Bromo-2-chloro-N-Fmoc-L-Phe .
- The diazomethylene group in this compound may offer unique reactivity under specific conditions (e.g., photolysis or acidolysis), though this remains less explored than the acetyloxy cleavable linker in Fmoc-Gly-NH-CH₂-acetyloxy (CAS: 1599440-06-8) .
Steric and Conformational Impact
- Glycine derivatives like this compound and Fmoc-Gly-OH are preferred in SPPS for minimizing steric clashes during peptide elongation . In contrast, bulkier residues (e.g., Fmoc-2-fluoro-N-Me-L-Phe ) introduce rigidity, altering peptide secondary structures .
Data Tables
Table 1: Physicochemical Properties
*Exact solubility data for this compound requires further validation .
Table 2: Market Availability
Supplier | This compound | Fmoc-Gly-OH | Fmoc-Glu(OtBu)-Gly-OH |
---|---|---|---|
Absin | Yes | No | No |
Glentham Life Sciences | Yes | Yes | No |
Advanced ChemTech | No | Yes | Yes |
Activité Biologique
Fmoc-Gly-CHN2 (CAS No. 275816-73-4) is a derivative of glycine protected by the Fmoc (9-fluorenylmethoxycarbonyl) group, which is widely utilized in peptide synthesis and proteomics. This compound exhibits notable biological activities primarily through its role as a protease inhibitor, particularly affecting cysteine proteases such as cathepsins.
The biological activity of this compound is largely attributed to its ability to form covalent bonds with the active sites of specific proteases. This irreversible inhibition alters the normal function of these enzymes, leading to various cellular responses, including apoptosis in certain cancer cell lines.
Inhibition of Cysteine Proteases
Research indicates that this compound and its analogs effectively inhibit cathepsins B and L, which are crucial for protein turnover and degradation within cells. The inhibition mechanism involves the formation of stable covalent bonds with the catalytic residues of these enzymes, thereby preventing their activity.
Table 1: Inhibition Potency of this compound and Related Compounds
Compound | Target Enzyme | IC50 (nM) | Specificity |
---|---|---|---|
This compound | Cathepsin B | TBD | High |
Fmoc-Tyr-Ala-CHN2 | Cathepsin B & L | ~100 | Selective for neuroblastoma cells |
Z-Phe-Ala-CHN2 | Cathepsin L | TBD | Low |
Notes :
- The IC50 value for this compound is yet to be determined (TBD) in specific studies.
- Fmoc-Tyr-Ala-CHN2 has demonstrated significant selectivity towards neuroblastoma cells, indicating potential therapeutic applications.
Neuroblastoma Research
A study highlighted the effects of protease inhibitors on neuroblastoma cells, where Fmoc-Tyr-Ala-CHN2 induced apoptosis selectively in these cells without affecting other cancer types. This specificity is crucial for developing targeted cancer therapies that minimize damage to healthy tissues .
Mechanistic Insights
In another investigation, it was observed that treatment with Fmoc-Tyr-Ala-CHN2 led to the accumulation of electron-dense vesicles within neuroblastoma cells, suggesting impaired lysosomal function due to cathepsin inhibition. The study concluded that a significant reduction in cathepsin activity (greater than 90%) was necessary to observe measurable effects on cell growth and viability .
Implications for Therapeutic Development
The selective inhibition of cathepsins by compounds like this compound opens avenues for developing novel therapeutic agents against cancers characterized by elevated levels of these proteases. The specificity towards neuroblastoma indicates potential for reducing side effects commonly associated with broader-spectrum chemotherapeutics.
Q & A
Q. What are the key considerations for synthesizing Fmoc-Gly-CHN2 with high purity?
Basic Question
To achieve high-purity this compound, focus on:
- Reaction Conditions : Use anhydrous solvents (e.g., DMF or DCM) and inert atmospheres to prevent hydrolysis of the diazomethane (CHN2) group.
- Protection/Deprotection : Ensure complete Fmoc protection of the glycine amine group while avoiding over-activation of the coupling reagent, which can lead to side reactions.
- Purification : Employ reverse-phase HPLC or flash chromatography for isolation, followed by recrystallization in non-polar solvents (e.g., hexane/ethyl acetate mixtures) .
- Analytical Validation : Confirm purity via NMR (e.g., absence of residual solvents in H NMR) and mass spectrometry (MS) for molecular ion verification .
Q. Which analytical techniques are critical for confirming the structure and purity of this compound?
Basic Question
- Nuclear Magnetic Resonance (NMR) : H and C NMR to verify the Fmoc group (aromatic protons at 7.1–7.8 ppm) and glycine backbone (α-proton at ~4.0 ppm).
- Mass Spectrometry (MS) : High-resolution MS (HRMS) to confirm the molecular ion ([M+H]) and rule out adducts or degradation products.
- High-Performance Liquid Chromatography (HPLC) : Use a C18 column with UV detection (265 nm for Fmoc absorbance) to assess purity (>95% by area under the curve) .
Q. How can researchers address discrepancies in reported reactivity of this compound under different coupling conditions?
Advanced Question
- Systematic Variation : Test coupling efficiency across solvents (DMF vs. DCM), bases (DIEA vs. NMM), and temperatures (0°C vs. RT).
- Statistical Analysis : Use ANOVA to compare yields under varying conditions, ensuring replicates (n ≥ 3) to account for experimental error .
- Literature Triangulation : Cross-reference protocols from peer-reviewed journals (via Google Scholar’s citation ranking) to identify consensus methodologies .
Q. What strategies optimize the stability of this compound during long-term storage?
Advanced Question
- Storage Conditions : Store at –20°C in airtight, light-protected vials with desiccants (e.g., silica gel). Avoid repeated freeze-thaw cycles.
- Stability Assays : Periodically analyze samples via HPLC to monitor degradation (e.g., hydrolysis to Fmoc-Gly-OH).
- Batch Documentation : Maintain detailed logs of storage duration and conditions, adhering to FAIR data principles for reproducibility .
Q. What are the primary applications of this compound in peptide synthesis methodologies?
Basic Question
- Solid-Phase Peptide Synthesis (SPPS) : Used as a glycine derivative for coupling to resin-bound peptides. The CHN2 group facilitates carbodiimide-mediated activation.
- Kinetic Studies : Monitor coupling rates via UV monitoring of Fmoc deprotection (piperidine cleavage) to optimize elongation efficiency .
Q. How should researchers design experiments to evaluate the kinetic parameters of this compound in peptide chain elongation?
Advanced Question
- Experimental Design :
- Variables : Vary reagent concentration, temperature, and coupling time.
- Controls : Include a reference amino acid (e.g., Fmoc-Ala-OH) to benchmark reactivity.
- Data Collection : Use inline IR spectroscopy to track carbodiimide consumption or HPLC to quantify unreacted starting material.
- FINER Criteria : Ensure feasibility (lab resources), novelty (comparison to existing data), and relevance (impact on SPPS optimization) .
Q. What are common by-products formed during this compound activation, and how can they be identified?
Basic Question
- By-Products :
- Diazomethane Derivatives : Degradation to methyl esters under acidic conditions.
- Dipeptides : Due to premature coupling or racemization.
- Identification :
Q. How can computational modeling be integrated with experimental data to predict this compound reactivity?
Advanced Question
- Molecular Dynamics (MD) : Simulate solvent interactions to predict solubility and aggregation tendencies.
- Density Functional Theory (DFT) : Calculate activation energies for coupling reactions to identify rate-limiting steps.
- Validation : Compare computational predictions with experimental kinetic data (e.g., Arrhenius plots). Share models via repositories like Chemotion-ELN for community validation .
Q. Notes on Methodology and Citations
- Data Management : Adhere to NIH guidelines for experimental reporting (e.g., detailed protocols in supplementary materials) .
- Literature Review : Use Google Scholar’s citation metrics to prioritize high-impact studies .
- Ethical Compliance : Address conflicts in data through peer consultation and transparent documentation .
Propriétés
IUPAC Name |
9H-fluoren-9-ylmethyl N-(3-diazo-2-oxopropyl)carbamate | |
---|---|---|
Source | PubChem | |
URL | https://pubchem.ncbi.nlm.nih.gov | |
Description | Data deposited in or computed by PubChem | |
InChI |
InChI=1S/C18H15N3O3/c19-21-10-12(22)9-20-18(23)24-11-17-15-7-3-1-5-13(15)14-6-2-4-8-16(14)17/h1-8,10,17H,9,11H2,(H,20,23) | |
Source | PubChem | |
URL | https://pubchem.ncbi.nlm.nih.gov | |
Description | Data deposited in or computed by PubChem | |
InChI Key |
DLDGJOVHMQKZMI-UHFFFAOYSA-N | |
Source | PubChem | |
URL | https://pubchem.ncbi.nlm.nih.gov | |
Description | Data deposited in or computed by PubChem | |
Canonical SMILES |
C1=CC=C2C(=C1)C(C3=CC=CC=C32)COC(=O)NCC(=O)C=[N+]=[N-] | |
Source | PubChem | |
URL | https://pubchem.ncbi.nlm.nih.gov | |
Description | Data deposited in or computed by PubChem | |
Molecular Formula |
C18H15N3O3 | |
Source | PubChem | |
URL | https://pubchem.ncbi.nlm.nih.gov | |
Description | Data deposited in or computed by PubChem | |
Molecular Weight |
321.3 g/mol | |
Source | PubChem | |
URL | https://pubchem.ncbi.nlm.nih.gov | |
Description | Data deposited in or computed by PubChem | |
Avertissement et informations sur les produits de recherche in vitro
Veuillez noter que tous les articles et informations sur les produits présentés sur BenchChem sont destinés uniquement à des fins informatives. Les produits disponibles à l'achat sur BenchChem sont spécifiquement conçus pour des études in vitro, qui sont réalisées en dehors des organismes vivants. Les études in vitro, dérivées du terme latin "in verre", impliquent des expériences réalisées dans des environnements de laboratoire contrôlés à l'aide de cellules ou de tissus. Il est important de noter que ces produits ne sont pas classés comme médicaments et n'ont pas reçu l'approbation de la FDA pour la prévention, le traitement ou la guérison de toute condition médicale, affection ou maladie. Nous devons souligner que toute forme d'introduction corporelle de ces produits chez les humains ou les animaux est strictement interdite par la loi. Il est essentiel de respecter ces directives pour assurer la conformité aux normes légales et éthiques en matière de recherche et d'expérimentation.