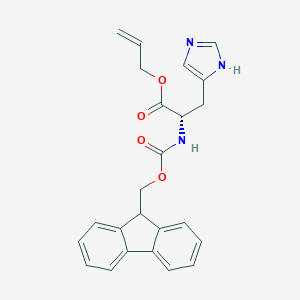
Fmoc-L-his-oall
Vue d'ensemble
Description
Fmoc-L-histidine allyl ester: is a derivative of the amino acid histidine, modified with a fluorenylmethyloxycarbonyl (Fmoc) protecting group at the N-terminus and an allyl ester group at the C-terminus. This compound is primarily used in peptide synthesis, where the Fmoc group serves as a temporary protecting group for the amino group, allowing for the stepwise construction of peptides on a solid support. The allyl ester group can be selectively removed under mild conditions, making it a versatile tool in the synthesis of complex peptides and proteins .
Mécanisme D'action
Target of Action
The primary target of Fmoc-L-his-oall is the Nα-amino group of an amino acid during peptide synthesis . The compound is used as a protecting group for the Nα-amino group, which is a crucial step in the chemical formation of the peptide bond .
Mode of Action
This compound operates by protecting the Nα-amino group of an amino acid during the peptide synthesis process . This protection is necessary for the activation of the carboxyl group of an amino acid, which is a key step in the formation of the peptide bond . The Fmoc group can be introduced by reacting the amine with fluorenylmethyloxycarbonyl chloride (Fmoc-Cl) .
Biochemical Pathways
The use of this compound is integral to the chemical synthesis of peptides , particularly in the method known as solid-phase peptide synthesis (SPPS) . This process involves the step-by-step assembly of the peptide chain, with each amino acid added one at a time. The Fmoc group allows for the rapid and efficient synthesis of peptides, including those of significant size and complexity .
Pharmacokinetics
It’s worth noting that the fmoc group is stable to treatment with trifluoroacetic acid (tfa) and hydrogen bromide/acetic acid .
Result of Action
The use of this compound results in the successful synthesis of peptides . By protecting the Nα-amino group, this compound allows for the efficient formation of peptide bonds, enabling the creation of complex peptides .
Action Environment
The action of this compound is influenced by the conditions of the peptide synthesis process. For instance, the removal of the Fmoc group is achieved with a solution of 20% piperidine in N,N-dimethylformamide (DMF) . The stability of the Fmoc group to various treatments also contributes to its efficacy in different environments .
Méthodes De Préparation
Synthetic Routes and Reaction Conditions: The synthesis of Fmoc-L-histidine allyl ester typically involves the following steps:
Protection of the amino group: The amino group of L-histidine is protected with the Fmoc group using Fmoc chloride in the presence of a base such as sodium carbonate or triethylamine.
Esterification: The carboxyl group of the Fmoc-protected histidine is then esterified with allyl alcohol in the presence of a coupling reagent such as dicyclohexylcarbodiimide (DCC) and a catalyst like 4-dimethylaminopyridine (DMAP).
Industrial Production Methods: In an industrial setting, the production of Fmoc-L-histidine allyl ester follows similar steps but on a larger scale. The process involves:
Large-scale protection: Using automated systems to protect the amino group with Fmoc chloride.
Bulk esterification: Conducting the esterification reaction in large reactors with continuous monitoring and control of reaction conditions to ensure high yield and purity
Analyse Des Réactions Chimiques
Types of Reactions: Fmoc-L-histidine allyl ester undergoes several types of chemical reactions, including:
Deprotection: Removal of the Fmoc group using a base such as piperidine.
Ester hydrolysis: Cleavage of the allyl ester group using palladium catalysts in the presence of a nucleophile like morpholine.
Common Reagents and Conditions:
Deprotection: Piperidine in dimethylformamide (DMF) at room temperature.
Ester hydrolysis: Palladium(0) catalysts in the presence of morpholine or other nucleophiles.
Major Products:
Deprotection: Yields L-histidine with a free amino group.
Ester hydrolysis: Produces L-histidine with a free carboxyl group
Applications De Recherche Scientifique
Chemistry: Fmoc-L-histidine allyl ester is widely used in the synthesis of peptides and proteins. Its protecting groups allow for selective deprotection and modification, facilitating the construction of complex peptide sequences.
Biology: In biological research, this compound is used to study protein-protein interactions and enzyme-substrate interactions. It serves as a building block for the synthesis of peptide-based inhibitors and probes.
Medicine: Fmoc-L-histidine allyl ester is utilized in the development of peptide-based therapeutics. Its ability to be selectively deprotected makes it valuable in the synthesis of drug candidates with specific functional groups.
Industry: In the pharmaceutical and biotechnology industries, this compound is used in the large-scale synthesis of peptides and proteins for therapeutic and diagnostic applications .
Comparaison Avec Des Composés Similaires
Fmoc-L-histidine (trityl): Similar to Fmoc-L-histidine allyl ester but with a trityl protecting group instead of an allyl ester.
Fmoc-L-histidine (Boc): Uses a tert-butyloxycarbonyl (Boc) group for protection.
Fmoc-L-histidine (OtBu): Contains a tert-butyl ester group for protection.
Uniqueness: Fmoc-L-histidine allyl ester is unique due to its combination of Fmoc and allyl ester protecting groups. This allows for selective deprotection under mild conditions, making it highly versatile in peptide synthesis. The allyl ester group can be removed without affecting other protecting groups, providing greater control over the synthesis process .
Activité Biologique
Fmoc-L-his-oall is a derivative of histidine, an essential amino acid known for its role in various biological processes. The Fmoc (9-fluorenylmethoxycarbonyl) group is commonly used in peptide synthesis to protect the amino group of the amino acid during synthesis. This article focuses on the biological activity of this compound, highlighting its interactions, mechanisms, and potential applications based on recent research findings.
This compound is characterized by its unique structure, which includes:
- Fmoc Group : Provides stability and protection during synthesis.
- Histidine Residue : Contains an imidazole side chain, contributing to its catalytic and binding properties.
Enzymatic Activity
Recent studies have demonstrated that this compound can modulate the assembly behavior of peptide nanomaterials, particularly in the context of amyloid-like structures. Histidine's imidazole group plays a crucial role in facilitating enzyme-like catalysis, particularly in generating reactive oxygen species (ROS) from assembled peptide structures. This catalytic behavior mimics peroxidase activity, which has implications for both therapeutic and toxicological studies involving neurodegenerative diseases such as Alzheimer's disease (AD) .
- Catalytic Mechanism : The imidazole group of histidine acts as an active site for catalyzing reactions that produce ROS, leading to potential cellular toxicity and damage .
Peptide Assembly
The presence of histidine in peptide assemblies has been shown to influence their structural properties significantly. For instance, this compound promotes the aggregation of dipeptides into nanofilaments through electrostatic interactions and hydrogen bonding. This process is critical for developing novel nanomaterials with unique properties suitable for biomedical applications .
Property | Description |
---|---|
Peptide Assembly Type | Nanofilament formation |
Interaction Mechanism | Electrostatic interactions, hydrogen bonding |
Catalytic Activity | Mimics peroxidase-like activity |
Neurotoxicity Studies
In vivo experiments have indicated that peptides assembled from this compound can induce ROS toxicity, damaging neuronal cells. This finding is particularly relevant for understanding the mechanisms underlying AD pathogenesis, where amyloid-beta (Aβ) aggregation leads to neurodegeneration .
Study 1: Amyloid-like Assembly Modulation
A study investigated how this compound influences the assembly of Fmoc-dipeptides into amyloid-like structures. The results showed that histidine significantly enhances fibrillogenesis compared to other amino acids, suggesting its pivotal role in forming stable peptide aggregates .
Study 2: Toxicity Assessment
Another research focused on the neurotoxic effects of Aβ filaments formed in the presence of this compound. It was observed that prolonged exposure to these assemblies resulted in increased ROS generation and subsequent neuronal damage, providing insights into the potential risks associated with peptide-based therapies targeting neurodegenerative diseases .
Research Findings Summary
- This compound exhibits significant catalytic activity , mimicking peroxidase enzymes.
- Promotes the formation of amyloid-like structures , which may have implications for developing nanomaterials.
- Induces neurotoxicity through ROS generation , linking it to neurodegenerative disease mechanisms.
Propriétés
IUPAC Name |
prop-2-enyl (2S)-2-(9H-fluoren-9-ylmethoxycarbonylamino)-3-(1H-imidazol-5-yl)propanoate | |
---|---|---|
Source | PubChem | |
URL | https://pubchem.ncbi.nlm.nih.gov | |
Description | Data deposited in or computed by PubChem | |
InChI |
InChI=1S/C24H23N3O4/c1-2-11-30-23(28)22(12-16-13-25-15-26-16)27-24(29)31-14-21-19-9-5-3-7-17(19)18-8-4-6-10-20(18)21/h2-10,13,15,21-22H,1,11-12,14H2,(H,25,26)(H,27,29)/t22-/m0/s1 | |
Source | PubChem | |
URL | https://pubchem.ncbi.nlm.nih.gov | |
Description | Data deposited in or computed by PubChem | |
InChI Key |
SDJWDDVYGPTKBQ-QFIPXVFZSA-N | |
Source | PubChem | |
URL | https://pubchem.ncbi.nlm.nih.gov | |
Description | Data deposited in or computed by PubChem | |
Canonical SMILES |
C=CCOC(=O)C(CC1=CN=CN1)NC(=O)OCC2C3=CC=CC=C3C4=CC=CC=C24 | |
Source | PubChem | |
URL | https://pubchem.ncbi.nlm.nih.gov | |
Description | Data deposited in or computed by PubChem | |
Isomeric SMILES |
C=CCOC(=O)[C@H](CC1=CN=CN1)NC(=O)OCC2C3=CC=CC=C3C4=CC=CC=C24 | |
Source | PubChem | |
URL | https://pubchem.ncbi.nlm.nih.gov | |
Description | Data deposited in or computed by PubChem | |
Molecular Formula |
C24H23N3O4 | |
Source | PubChem | |
URL | https://pubchem.ncbi.nlm.nih.gov | |
Description | Data deposited in or computed by PubChem | |
Molecular Weight |
417.5 g/mol | |
Source | PubChem | |
URL | https://pubchem.ncbi.nlm.nih.gov | |
Description | Data deposited in or computed by PubChem | |
Retrosynthesis Analysis
AI-Powered Synthesis Planning: Our tool employs the Template_relevance Pistachio, Template_relevance Bkms_metabolic, Template_relevance Pistachio_ringbreaker, Template_relevance Reaxys, Template_relevance Reaxys_biocatalysis model, leveraging a vast database of chemical reactions to predict feasible synthetic routes.
One-Step Synthesis Focus: Specifically designed for one-step synthesis, it provides concise and direct routes for your target compounds, streamlining the synthesis process.
Accurate Predictions: Utilizing the extensive PISTACHIO, BKMS_METABOLIC, PISTACHIO_RINGBREAKER, REAXYS, REAXYS_BIOCATALYSIS database, our tool offers high-accuracy predictions, reflecting the latest in chemical research and data.
Strategy Settings
Precursor scoring | Relevance Heuristic |
---|---|
Min. plausibility | 0.01 |
Model | Template_relevance |
Template Set | Pistachio/Bkms_metabolic/Pistachio_ringbreaker/Reaxys/Reaxys_biocatalysis |
Top-N result to add to graph | 6 |
Feasible Synthetic Routes
Avertissement et informations sur les produits de recherche in vitro
Veuillez noter que tous les articles et informations sur les produits présentés sur BenchChem sont destinés uniquement à des fins informatives. Les produits disponibles à l'achat sur BenchChem sont spécifiquement conçus pour des études in vitro, qui sont réalisées en dehors des organismes vivants. Les études in vitro, dérivées du terme latin "in verre", impliquent des expériences réalisées dans des environnements de laboratoire contrôlés à l'aide de cellules ou de tissus. Il est important de noter que ces produits ne sont pas classés comme médicaments et n'ont pas reçu l'approbation de la FDA pour la prévention, le traitement ou la guérison de toute condition médicale, affection ou maladie. Nous devons souligner que toute forme d'introduction corporelle de ces produits chez les humains ou les animaux est strictement interdite par la loi. Il est essentiel de respecter ces directives pour assurer la conformité aux normes légales et éthiques en matière de recherche et d'expérimentation.