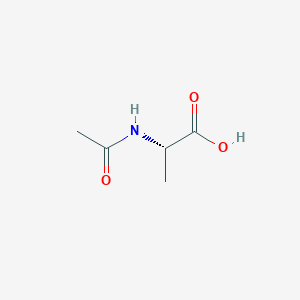
N-Acétyl-L-alanine
Vue d'ensemble
Description
N-Acetyl-L-alanine is an N-acylated alpha-amino acid where the L-alanine molecule is acetylated at the nitrogen atom. This compound is known for its role as a metabolite in various biological processes and has been identified as a biomarker in several studies, including those related to COVID-19 .
Applications De Recherche Scientifique
N-Acetyl-L-alanine has a wide range of applications in scientific research:
Chemistry: Used as a substrate for the identification and characterization of aminoacylase enzymes.
Biology: Acts as a metabolite in human and yeast metabolism, playing a role in various biochemical pathways.
Medicine: Identified as a biomarker for diseases such as COVID-19 and HIV, where it affects immune responses
Industry: Utilized in the synthesis of peptides and other bioactive compounds.
Mécanisme D'action
Target of Action
N-Acetyl-L-alanine primarily targets the Aldose reductase enzyme in humans . Aldose reductase is an enzyme involved in the polyol pathway, a two-step process that converts glucose to fructose. This enzyme plays a crucial role in various metabolic processes and diseases, including diabetic complications.
Mode of Action
It’s known that n-acetyl-l-alanine can act as a substrate for various enzymes such as aminoacylases and amidohydrolases . These enzymes could potentially modulate the activity of Aldose reductase, thereby influencing the metabolic processes it’s involved in.
Biochemical Pathways
N-Acetyl-L-alanine is classified as an N-acyl-L-alpha-amino acid . These are N-acylated alpha amino acids which have the L-configuration of the alpha-carbon atom .
Pharmacokinetics
Therefore, the impact of these properties on the bioavailability of N-Acetyl-L-alanine is currently unknown .
Result of Action
Given its potential interaction with aldose reductase, it may influence the metabolic processes regulated by this enzyme .
Analyse Biochimique
Biochemical Properties
N-Acetyl-L-alanine plays a significant role in biochemical reactions, particularly in the context of amino acid metabolism. It serves as a substrate for enzymes such as aminoacylase and amidohydrolase . These enzymes catalyze the hydrolysis of N-acetylated amino acids, releasing free amino acids and acetate. The interaction between N-Acetyl-L-alanine and these enzymes is crucial for the regulation of amino acid levels in cells and tissues.
Cellular Effects
N-Acetyl-L-alanine influences various cellular processes, including cell signaling pathways, gene expression, and cellular metabolism. It has been observed to affect the activity of certain enzymes involved in metabolic pathways, thereby altering the flux of metabolites within the cell . Additionally, N-Acetyl-L-alanine can modulate gene expression by interacting with transcription factors and other regulatory proteins, leading to changes in cellular function and behavior.
Molecular Mechanism
The molecular mechanism of N-Acetyl-L-alanine involves its interaction with specific biomolecules, such as enzymes and proteins. It binds to the active sites of aminoacylase and amidohydrolase, facilitating the hydrolysis of the acetyl group from the alanine molecule . This interaction can result in the activation or inhibition of these enzymes, depending on the cellular context and the presence of other regulatory factors. Furthermore, N-Acetyl-L-alanine can influence gene expression by binding to transcription factors and altering their activity.
Temporal Effects in Laboratory Settings
In laboratory settings, the effects of N-Acetyl-L-alanine can change over time due to its stability and degradation. Studies have shown that N-Acetyl-L-alanine is relatively stable under physiological conditions, but it can undergo hydrolysis over extended periods . This degradation can lead to a gradual decrease in its concentration and, consequently, its effects on cellular function. Long-term studies have also indicated that prolonged exposure to N-Acetyl-L-alanine can result in adaptive changes in cellular metabolism and gene expression.
Dosage Effects in Animal Models
The effects of N-Acetyl-L-alanine vary with different dosages in animal models. At low doses, it can enhance cellular function and metabolic activity, while at high doses, it may exhibit toxic or adverse effects . Threshold effects have been observed, where a certain concentration of N-Acetyl-L-alanine is required to elicit a significant biological response. Beyond this threshold, further increases in dosage can lead to diminishing returns or negative outcomes.
Metabolic Pathways
N-Acetyl-L-alanine is involved in several metabolic pathways, including amino acid metabolism and the acetylation cycle. It interacts with enzymes such as aminoacylase and amidohydrolase, which catalyze its hydrolysis and release free alanine and acetate . These metabolic reactions are essential for maintaining the balance of amino acids and acetyl groups within the cell, influencing overall metabolic flux and metabolite levels.
Transport and Distribution
Within cells and tissues, N-Acetyl-L-alanine is transported and distributed through specific transporters and binding proteins. These molecules facilitate its movement across cellular membranes and its localization to specific cellular compartments . The distribution of N-Acetyl-L-alanine can affect its concentration and activity in different regions of the cell, influencing its overall biological effects.
Subcellular Localization
N-Acetyl-L-alanine is localized to various subcellular compartments, including the cytoplasm and organelles such as mitochondria and lysosomes . Its subcellular localization is determined by targeting signals and post-translational modifications that direct it to specific regions within the cell. This localization can impact its activity and function, as it interacts with different biomolecules in distinct cellular environments.
Méthodes De Préparation
Synthetic Routes and Reaction Conditions: N-Acetyl-L-alanine can be synthesized through the acetylation of L-alanine. One common method involves reacting L-alanine with acetic anhydride in the presence of a base such as pyridine. The reaction typically proceeds at room temperature, yielding N-Acetyl-L-alanine after purification .
Industrial Production Methods: In an industrial setting, N-Acetyl-L-alanine can be produced by reacting L-alanine with acetyl chloride in a solvent like toluene. The reaction is carried out at elevated temperatures, followed by cooling and filtration to obtain the product with high purity .
Analyse Des Réactions Chimiques
Types of Reactions: N-Acetyl-L-alanine undergoes various chemical reactions, including:
Oxidation: It can be oxidized to form corresponding oxoacids.
Reduction: It can be reduced to form amines.
Substitution: It can participate in nucleophilic substitution reactions where the acetyl group can be replaced by other functional groups
Common Reagents and Conditions:
Oxidation: Common oxidizing agents include potassium permanganate and hydrogen peroxide.
Reduction: Reducing agents like lithium aluminum hydride are used.
Substitution: Reagents such as alkyl halides can be used under basic conditions
Major Products:
Oxidation: Produces oxoacids.
Reduction: Produces amines.
Substitution: Produces various substituted derivatives depending on the reagent used
Comparaison Avec Des Composés Similaires
N-Acetyl-L-alanine is unique among N-acylated amino acids due to its specific acetylation at the nitrogen atom of L-alanine. Similar compounds include:
- N-Acetyl-L-methionine
- N-Acetyl-L-proline
- N-Acetyl-L-cysteine
- N-Acetyl-L-asparagine
These compounds share the N-acylation but differ in the amino acid component, leading to variations in their biochemical properties and applications .
Propriétés
IUPAC Name |
2-acetamidopropanoic acid | |
---|---|---|
Source | PubChem | |
URL | https://pubchem.ncbi.nlm.nih.gov | |
Description | Data deposited in or computed by PubChem | |
InChI |
InChI=1S/C5H9NO3/c1-3(5(8)9)6-4(2)7/h3H,1-2H3,(H,6,7)(H,8,9) | |
Source | PubChem | |
URL | https://pubchem.ncbi.nlm.nih.gov | |
Description | Data deposited in or computed by PubChem | |
InChI Key |
KTHDTJVBEPMMGL-UHFFFAOYSA-N | |
Source | PubChem | |
URL | https://pubchem.ncbi.nlm.nih.gov | |
Description | Data deposited in or computed by PubChem | |
Canonical SMILES |
CC(C(=O)O)NC(=O)C | |
Source | PubChem | |
URL | https://pubchem.ncbi.nlm.nih.gov | |
Description | Data deposited in or computed by PubChem | |
Molecular Formula |
C5H9NO3 | |
Source | PubChem | |
URL | https://pubchem.ncbi.nlm.nih.gov | |
Description | Data deposited in or computed by PubChem | |
Molecular Weight |
131.13 g/mol | |
Source | PubChem | |
URL | https://pubchem.ncbi.nlm.nih.gov | |
Description | Data deposited in or computed by PubChem | |
CAS No. |
1115-69-1, 19436-52-3, 97-69-8 | |
Record name | N-Acetylalanine | |
Source | CAS Common Chemistry | |
URL | https://commonchemistry.cas.org/detail?cas_rn=1115-69-1 | |
Description | CAS Common Chemistry is an open community resource for accessing chemical information. Nearly 500,000 chemical substances from CAS REGISTRY cover areas of community interest, including common and frequently regulated chemicals, and those relevant to high school and undergraduate chemistry classes. This chemical information, curated by our expert scientists, is provided in alignment with our mission as a division of the American Chemical Society. | |
Explanation | The data from CAS Common Chemistry is provided under a CC-BY-NC 4.0 license, unless otherwise stated. | |
Record name | N-Acetyl-DL-alanine | |
Source | ChemIDplus | |
URL | https://pubchem.ncbi.nlm.nih.gov/substance/?source=chemidplus&sourceid=0001115691 | |
Description | ChemIDplus is a free, web search system that provides access to the structure and nomenclature authority files used for the identification of chemical substances cited in National Library of Medicine (NLM) databases, including the TOXNET system. | |
Record name | NSC203819 | |
Source | DTP/NCI | |
URL | https://dtp.cancer.gov/dtpstandard/servlet/dwindex?searchtype=NSC&outputformat=html&searchlist=203819 | |
Description | The NCI Development Therapeutics Program (DTP) provides services and resources to the academic and private-sector research communities worldwide to facilitate the discovery and development of new cancer therapeutic agents. | |
Explanation | Unless otherwise indicated, all text within NCI products is free of copyright and may be reused without our permission. Credit the National Cancer Institute as the source. | |
Record name | Acetylalanine | |
Source | DTP/NCI | |
URL | https://dtp.cancer.gov/dtpstandard/servlet/dwindex?searchtype=NSC&outputformat=html&searchlist=186892 | |
Description | The NCI Development Therapeutics Program (DTP) provides services and resources to the academic and private-sector research communities worldwide to facilitate the discovery and development of new cancer therapeutic agents. | |
Explanation | Unless otherwise indicated, all text within NCI products is free of copyright and may be reused without our permission. Credit the National Cancer Institute as the source. | |
Record name | 1115-69-1 | |
Source | DTP/NCI | |
URL | https://dtp.cancer.gov/dtpstandard/servlet/dwindex?searchtype=NSC&outputformat=html&searchlist=43118 | |
Description | The NCI Development Therapeutics Program (DTP) provides services and resources to the academic and private-sector research communities worldwide to facilitate the discovery and development of new cancer therapeutic agents. | |
Explanation | Unless otherwise indicated, all text within NCI products is free of copyright and may be reused without our permission. Credit the National Cancer Institute as the source. | |
Record name | N-acetyl-DL-alanine | |
Source | European Chemicals Agency (ECHA) | |
URL | https://echa.europa.eu/substance-information/-/substanceinfo/100.012.936 | |
Description | The European Chemicals Agency (ECHA) is an agency of the European Union which is the driving force among regulatory authorities in implementing the EU's groundbreaking chemicals legislation for the benefit of human health and the environment as well as for innovation and competitiveness. | |
Explanation | Use of the information, documents and data from the ECHA website is subject to the terms and conditions of this Legal Notice, and subject to other binding limitations provided for under applicable law, the information, documents and data made available on the ECHA website may be reproduced, distributed and/or used, totally or in part, for non-commercial purposes provided that ECHA is acknowledged as the source: "Source: European Chemicals Agency, http://echa.europa.eu/". Such acknowledgement must be included in each copy of the material. ECHA permits and encourages organisations and individuals to create links to the ECHA website under the following cumulative conditions: Links can only be made to webpages that provide a link to the Legal Notice page. | |
Retrosynthesis Analysis
AI-Powered Synthesis Planning: Our tool employs the Template_relevance Pistachio, Template_relevance Bkms_metabolic, Template_relevance Pistachio_ringbreaker, Template_relevance Reaxys, Template_relevance Reaxys_biocatalysis model, leveraging a vast database of chemical reactions to predict feasible synthetic routes.
One-Step Synthesis Focus: Specifically designed for one-step synthesis, it provides concise and direct routes for your target compounds, streamlining the synthesis process.
Accurate Predictions: Utilizing the extensive PISTACHIO, BKMS_METABOLIC, PISTACHIO_RINGBREAKER, REAXYS, REAXYS_BIOCATALYSIS database, our tool offers high-accuracy predictions, reflecting the latest in chemical research and data.
Strategy Settings
Precursor scoring | Relevance Heuristic |
---|---|
Min. plausibility | 0.01 |
Model | Template_relevance |
Template Set | Pistachio/Bkms_metabolic/Pistachio_ringbreaker/Reaxys/Reaxys_biocatalysis |
Top-N result to add to graph | 6 |
Feasible Synthetic Routes
Q1: What is the molecular formula and weight of N-Acetyl-L-alanine?
A1: The molecular formula of N-Acetyl-L-alanine is C5H9NO3 and its molecular weight is 131.13 g/mol. []
Q2: What spectroscopic techniques are used to characterize N-Acetyl-L-alanine?
A2: Several spectroscopic techniques have been employed to characterize N-Acetyl-L-alanine, including:* Nuclear Magnetic Resonance (NMR): 1H and 13C NMR are used to determine the structure and study the interaction of N-Acetyl-L-alanine with solvents like trifluoroacetic acid. [, ]* Raman Spectroscopy: Provides insights into conformational equilibria in aqueous solutions under varying temperature and pressure. [] * Infrared (IR) Spectroscopy: IR spectroscopy, including vibrational circular dichroism (VCD), is used to analyze conformational changes and hydrogen bonding patterns. [, ]
Q3: What computational methods have been used to study N-Acetyl-L-alanine?
A3: Density functional theory (DFT) calculations, often at the B3LYP level, are widely used to study N-Acetyl-L-alanine. These calculations help predict vibrational frequencies, investigate hydration effects, and understand interactions with water molecules. [, , ]
Q4: Have there been any molecular dynamics simulations of N-Acetyl-L-alanine?
A4: Yes, molecular dynamics simulations using enhanced-sampling techniques like variationally enhanced sampling (VES) have been employed. These simulations help study conformational transitions and free energy landscapes of N-Acetyl-L-alanine, particularly in the context of dipeptide models. []
Q5: Is there information available on the stability of N-Acetyl-L-alanine under various conditions?
A5: While specific stability data is limited in the provided research, studies have investigated the heat capacity and phase behavior of N-Acetyl-L-alanine amide under different temperatures. [] This type of data can offer insights into the compound's stability across a temperature range.
Q6: How do structural modifications of N-Acetyl-L-alanine affect its biological activity?
A6: The provided research highlights the significance of the N-acetyl and L-alanine moieties for specific biological interactions. For example, replacing the sulfhydryl group in N-Acetyl-L-cysteine with the methyl group of N-Acetyl-L-alanine results in a loss of ciliostatic activity. [] This suggests that the sulfhydryl group is crucial for this particular effect.
Q7: What is the biological relevance of N-Acetyl-L-alanine?
A7: N-Acetyl-L-alanine is a simple N-acetylated amino acid. While not naturally occurring in high abundance, it serves as a valuable model compound in biochemical studies:* Model Peptide: It aids in understanding peptide bond characteristics, conformational preferences, and interactions with solvents. [, , ]* Substrate Analog: It's used to investigate enzyme specificity and kinetics, particularly for enzymes that act on N-acetylated peptides. [, ]* Metabolic Studies: It's investigated as a potential biomarker in metabolomic studies focusing on conditions like ovarian response to stimulation and bacterial infections. [, ]
Q8: Are there any known interactions of N-Acetyl-L-alanine with biological systems?
A8: While the research provided doesn't extensively detail specific interactions, one study shows that N-Acetyl-L-alanine is hydrolyzed at a slower rate than N-carbamoyl-L-alanine by the bacterial enzyme β-ureidopropionase from Pseudomonas putida IFO 12996. [, ] This highlights the enzyme's substrate preference and provides insight into its potential metabolic role.
Avertissement et informations sur les produits de recherche in vitro
Veuillez noter que tous les articles et informations sur les produits présentés sur BenchChem sont destinés uniquement à des fins informatives. Les produits disponibles à l'achat sur BenchChem sont spécifiquement conçus pour des études in vitro, qui sont réalisées en dehors des organismes vivants. Les études in vitro, dérivées du terme latin "in verre", impliquent des expériences réalisées dans des environnements de laboratoire contrôlés à l'aide de cellules ou de tissus. Il est important de noter que ces produits ne sont pas classés comme médicaments et n'ont pas reçu l'approbation de la FDA pour la prévention, le traitement ou la guérison de toute condition médicale, affection ou maladie. Nous devons souligner que toute forme d'introduction corporelle de ces produits chez les humains ou les animaux est strictement interdite par la loi. Il est essentiel de respecter ces directives pour assurer la conformité aux normes légales et éthiques en matière de recherche et d'expérimentation.