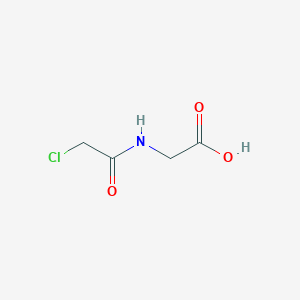
N-Chloroacetylglycine
Vue d'ensemble
Description
N-Chloroacetylglycine is an organic compound with the molecular formula C4H6ClNO3. It is a derivative of glycine, where the amino group is substituted with a chloroacetyl group. This compound is known for its white solid or crystalline appearance .
Méthodes De Préparation
Synthetic Routes and Reaction Conditions
N-Chloroacetylglycine can be synthesized through the reaction of glycine with chloroacetyl chloride in the presence of a base such as sodium hydroxide. The reaction is typically carried out in a two-phase system consisting of water and diethyl ether. The chloroacetyl chloride is added dropwise to the glycine solution at 0°C, and the mixture is stirred at this temperature for 30 minutes, followed by stirring at room temperature for an additional hour. The product is then extracted and purified .
Industrial Production Methods
While specific industrial production methods for this compound are not extensively documented, the general approach involves the same synthetic route as described above, with potential optimizations for scale-up, such as continuous flow reactors and automated systems to ensure consistent quality and yield.
Analyse Des Réactions Chimiques
Types of Reactions
N-Chloroacetylglycine primarily undergoes substitution reactions due to the presence of the chloroacetyl group. It can also participate in hydrolysis reactions under acidic or basic conditions.
Common Reagents and Conditions
Substitution Reactions: Common reagents include nucleophiles such as amines or thiols, which can replace the chlorine atom in the chloroacetyl group.
Hydrolysis Reactions: Acidic or basic conditions can lead to the hydrolysis of the chloroacetyl group, resulting in the formation of glycine and chloroacetic acid.
Major Products Formed
Substitution Reactions: The major products are derivatives of glycine where the chloroacetyl group is replaced by the nucleophile.
Hydrolysis Reactions: The major products are glycine and chloroacetic acid.
Applications De Recherche Scientifique
N-Chloroacetylglycine has several applications in scientific research:
Chemistry: It is used as an intermediate in the synthesis of various organic compounds, including peptides and polypeptides.
Biology: It serves as a building block in the study of enzyme mechanisms and protein modifications.
Medicine: It is investigated for its potential use in drug development, particularly in the design of enzyme inhibitors.
Industry: It is utilized in the production of specialty chemicals and as a reagent in analytical chemistry.
Mécanisme D'action
The mechanism of action of N-Chloroacetylglycine involves its ability to act as an acylating agent. The chloroacetyl group can react with nucleophilic sites on proteins and enzymes, leading to the modification of these biomolecules. This property makes it useful in the study of enzyme mechanisms and the development of enzyme inhibitors .
Comparaison Avec Des Composés Similaires
Similar Compounds
N-Acetylglycine: Similar to N-Chloroacetylglycine but with an acetyl group instead of a chloroacetyl group.
N-Formylglycine: Contains a formyl group instead of a chloroacetyl group.
N-Benzoylglycine: Contains a benzoyl group instead of a chloroacetyl group.
Uniqueness
This compound is unique due to the presence of the chloroacetyl group, which imparts distinct reactivity compared to other acylated glycine derivatives. This reactivity makes it particularly useful in substitution reactions and as an acylating agent in biochemical studies .
Activité Biologique
N-Chloroacetylglycine is a compound that has garnered attention in various fields of biological research due to its potential pharmacological applications and biochemical properties. This article provides a comprehensive overview of its biological activity, including synthesis, mechanisms of action, and relevant case studies.
Chemical Structure and Synthesis
This compound is an N-acyl derivative of glycine, characterized by the presence of a chloroacetyl group. Its synthesis typically involves the reaction of glycine with chloroacetyl chloride or chloroacetic acid under controlled conditions. The following reaction scheme illustrates its synthesis:
Enzymatic Hydrolysis
Research has shown that N-chloroacetylated amino acids, including this compound, can be substrates for various enzymes such as aminoacylases. These enzymes catalyze the hydrolysis of N-acyl amino acids, yielding corresponding organic acids and amino acids. A study involving Pyrococcus furiosus demonstrated that aminoacylase exhibits broad substrate specificity and can hydrolyze this compound effectively, suggesting its potential role in metabolic pathways involving detoxification and amino acid catabolism .
Neurotransmitter Modulation
This compound has been implicated in modulating neurotransmitter activity. Specifically, it has been observed to potentiate the activation of glycine receptors (GlyRs), which are crucial for inhibitory neurotransmission in the central nervous system. This modulation is significant as it may influence synaptic transmission and neuronal excitability. A study highlighted that N-acyl amino acids enhance glycine's activation of GlyRs, indicating a possible therapeutic avenue for conditions such as epilepsy .
Pharmacological Applications
- Opioid Research : this compound has been utilized in the design of haptens for vaccines against drug abuse. In this context, it was reacted with morphine derivatives to create compounds that could elicit an immune response against morphine, potentially aiding in addiction treatment .
- Antimicrobial Activity : Preliminary studies have suggested that derivatives of this compound may exhibit antimicrobial properties. For instance, a series of synthesized compounds based on this scaffold showed varying degrees of activity against bacterial strains, indicating its potential use in developing new antibiotics .
The biological activity of this compound can be attributed to several mechanisms:
- Enzyme Substrate Interaction : As a substrate for aminoacylases, it participates in metabolic processes that detoxify xenobiotic compounds.
- Neurotransmitter Receptor Modulation : By enhancing glycine receptor activity, it may play a role in regulating excitatory and inhibitory balance in neuronal circuits.
- Chemical Reactivity : The chloroacetyl group enhances its reactivity with nucleophiles, potentially leading to the formation of biologically active metabolites.
Data Summary
The following table summarizes key findings related to the biological activity of this compound:
Propriétés
IUPAC Name |
2-[(2-chloroacetyl)amino]acetic acid | |
---|---|---|
Source | PubChem | |
URL | https://pubchem.ncbi.nlm.nih.gov | |
Description | Data deposited in or computed by PubChem | |
InChI |
InChI=1S/C4H6ClNO3/c5-1-3(7)6-2-4(8)9/h1-2H2,(H,6,7)(H,8,9) | |
Source | PubChem | |
URL | https://pubchem.ncbi.nlm.nih.gov | |
Description | Data deposited in or computed by PubChem | |
InChI Key |
DLDTUYIGYMNERN-UHFFFAOYSA-N | |
Source | PubChem | |
URL | https://pubchem.ncbi.nlm.nih.gov | |
Description | Data deposited in or computed by PubChem | |
Canonical SMILES |
C(C(=O)O)NC(=O)CCl | |
Source | PubChem | |
URL | https://pubchem.ncbi.nlm.nih.gov | |
Description | Data deposited in or computed by PubChem | |
Molecular Formula |
C4H6ClNO3 | |
Source | PubChem | |
URL | https://pubchem.ncbi.nlm.nih.gov | |
Description | Data deposited in or computed by PubChem | |
DSSTOX Substance ID |
DTXSID70283545 | |
Record name | N-Chloroacetylglycine | |
Source | EPA DSSTox | |
URL | https://comptox.epa.gov/dashboard/DTXSID70283545 | |
Description | DSSTox provides a high quality public chemistry resource for supporting improved predictive toxicology. | |
Molecular Weight |
151.55 g/mol | |
Source | PubChem | |
URL | https://pubchem.ncbi.nlm.nih.gov | |
Description | Data deposited in or computed by PubChem | |
CAS No. |
6319-96-6 | |
Record name | 6319-96-6 | |
Source | DTP/NCI | |
URL | https://dtp.cancer.gov/dtpstandard/servlet/dwindex?searchtype=NSC&outputformat=html&searchlist=32036 | |
Description | The NCI Development Therapeutics Program (DTP) provides services and resources to the academic and private-sector research communities worldwide to facilitate the discovery and development of new cancer therapeutic agents. | |
Explanation | Unless otherwise indicated, all text within NCI products is free of copyright and may be reused without our permission. Credit the National Cancer Institute as the source. | |
Record name | N-Chloroacetylglycine | |
Source | EPA DSSTox | |
URL | https://comptox.epa.gov/dashboard/DTXSID70283545 | |
Description | DSSTox provides a high quality public chemistry resource for supporting improved predictive toxicology. | |
Retrosynthesis Analysis
AI-Powered Synthesis Planning: Our tool employs the Template_relevance Pistachio, Template_relevance Bkms_metabolic, Template_relevance Pistachio_ringbreaker, Template_relevance Reaxys, Template_relevance Reaxys_biocatalysis model, leveraging a vast database of chemical reactions to predict feasible synthetic routes.
One-Step Synthesis Focus: Specifically designed for one-step synthesis, it provides concise and direct routes for your target compounds, streamlining the synthesis process.
Accurate Predictions: Utilizing the extensive PISTACHIO, BKMS_METABOLIC, PISTACHIO_RINGBREAKER, REAXYS, REAXYS_BIOCATALYSIS database, our tool offers high-accuracy predictions, reflecting the latest in chemical research and data.
Strategy Settings
Precursor scoring | Relevance Heuristic |
---|---|
Min. plausibility | 0.01 |
Model | Template_relevance |
Template Set | Pistachio/Bkms_metabolic/Pistachio_ringbreaker/Reaxys/Reaxys_biocatalysis |
Top-N result to add to graph | 6 |
Feasible Synthetic Routes
Q1: What is the main chemical transformation explored in this research paper?
A1: The research investigates the reaction of N-Chloroacetylglycine ethyl ester with various hydrazines. [] This reaction leads to the formation of piperazine-2,5-dione derivatives, which are cyclic compounds with potential biological activities. The study explores how different substituents on the hydrazine molecule influence the reaction outcome and the properties of the resulting piperazine-2,5-diones.
Avertissement et informations sur les produits de recherche in vitro
Veuillez noter que tous les articles et informations sur les produits présentés sur BenchChem sont destinés uniquement à des fins informatives. Les produits disponibles à l'achat sur BenchChem sont spécifiquement conçus pour des études in vitro, qui sont réalisées en dehors des organismes vivants. Les études in vitro, dérivées du terme latin "in verre", impliquent des expériences réalisées dans des environnements de laboratoire contrôlés à l'aide de cellules ou de tissus. Il est important de noter que ces produits ne sont pas classés comme médicaments et n'ont pas reçu l'approbation de la FDA pour la prévention, le traitement ou la guérison de toute condition médicale, affection ou maladie. Nous devons souligner que toute forme d'introduction corporelle de ces produits chez les humains ou les animaux est strictement interdite par la loi. Il est essentiel de respecter ces directives pour assurer la conformité aux normes légales et éthiques en matière de recherche et d'expérimentation.