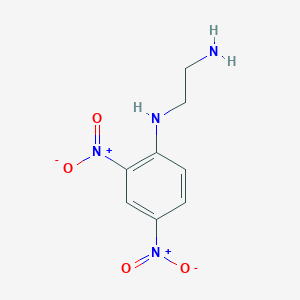
N-(2,4-Dinitrophenyl)ethylenediamine
Vue d'ensemble
Description
N-(2,4-Dinitrophenyl)ethylenediamine, also known as this compound, is a useful research compound. Its molecular formula is C8H10N4O4 and its molecular weight is 226.19 g/mol. The purity is usually 95%.
The exact mass of the compound this compound is unknown and the complexity rating of the compound is unknown. Its Medical Subject Headings (MeSH) category is Chemicals and Drugs Category - Organic Chemicals - Amines - Polyamines - Diamines - Ethylenediamines - Supplementary Records. The United Nations designated GHS hazard class pictogram is Irritant, and the GHS signal word is WarningThe storage condition is unknown. Please store according to label instructions upon receipt of goods.
BenchChem offers high-quality this compound suitable for many research applications. Different packaging options are available to accommodate customers' requirements. Please inquire for more information about this compound including the price, delivery time, and more detailed information at info@benchchem.com.
Mécanisme D'action
Mode of Action
It is known that many dinitrophenyl compounds interact with biological molecules through nucleophilic substitution reactions . In these reactions, a nucleophile (such as an amino group in a protein or peptide) replaces a nitro group in the dinitrophenyl compound, leading to the formation of a covalent bond .
Biochemical Pathways
Given its potential to form covalent bonds with amino groups, it may interact with a wide range of proteins and peptides, potentially affecting multiple biochemical pathways .
Propriétés
IUPAC Name |
N'-(2,4-dinitrophenyl)ethane-1,2-diamine | |
---|---|---|
Source | PubChem | |
URL | https://pubchem.ncbi.nlm.nih.gov | |
Description | Data deposited in or computed by PubChem | |
InChI |
InChI=1S/C8H10N4O4/c9-3-4-10-7-2-1-6(11(13)14)5-8(7)12(15)16/h1-2,5,10H,3-4,9H2 | |
Source | PubChem | |
URL | https://pubchem.ncbi.nlm.nih.gov | |
Description | Data deposited in or computed by PubChem | |
InChI Key |
AIUKPEQJKQUQKZ-UHFFFAOYSA-N | |
Source | PubChem | |
URL | https://pubchem.ncbi.nlm.nih.gov | |
Description | Data deposited in or computed by PubChem | |
Canonical SMILES |
C1=CC(=C(C=C1[N+](=O)[O-])[N+](=O)[O-])NCCN | |
Source | PubChem | |
URL | https://pubchem.ncbi.nlm.nih.gov | |
Description | Data deposited in or computed by PubChem | |
Molecular Formula |
C8H10N4O4 | |
Source | PubChem | |
URL | https://pubchem.ncbi.nlm.nih.gov | |
Description | Data deposited in or computed by PubChem | |
DSSTOX Substance ID |
DTXSID30182949 | |
Record name | N-(2,4-Dinitrophenyl)ethylenediamine | |
Source | EPA DSSTox | |
URL | https://comptox.epa.gov/dashboard/DTXSID30182949 | |
Description | DSSTox provides a high quality public chemistry resource for supporting improved predictive toxicology. | |
Molecular Weight |
226.19 g/mol | |
Source | PubChem | |
URL | https://pubchem.ncbi.nlm.nih.gov | |
Description | Data deposited in or computed by PubChem | |
CAS No. |
28767-75-1 | |
Record name | (2,4-Dinitrophenyl)ethylenediamine | |
Source | CAS Common Chemistry | |
URL | https://commonchemistry.cas.org/detail?cas_rn=28767-75-1 | |
Description | CAS Common Chemistry is an open community resource for accessing chemical information. Nearly 500,000 chemical substances from CAS REGISTRY cover areas of community interest, including common and frequently regulated chemicals, and those relevant to high school and undergraduate chemistry classes. This chemical information, curated by our expert scientists, is provided in alignment with our mission as a division of the American Chemical Society. | |
Explanation | The data from CAS Common Chemistry is provided under a CC-BY-NC 4.0 license, unless otherwise stated. | |
Record name | N-(2,4-Dinitrophenyl)ethylenediamine | |
Source | ChemIDplus | |
URL | https://pubchem.ncbi.nlm.nih.gov/substance/?source=chemidplus&sourceid=0028767751 | |
Description | ChemIDplus is a free, web search system that provides access to the structure and nomenclature authority files used for the identification of chemical substances cited in National Library of Medicine (NLM) databases, including the TOXNET system. | |
Record name | N-(2,4-Dinitrophenyl)ethylenediamine | |
Source | EPA DSSTox | |
URL | https://comptox.epa.gov/dashboard/DTXSID30182949 | |
Description | DSSTox provides a high quality public chemistry resource for supporting improved predictive toxicology. | |
Record name | N1-(2,4-Dinitro-phenyl)-ethane-1,2-diamine | |
Source | European Chemicals Agency (ECHA) | |
URL | https://echa.europa.eu/information-on-chemicals | |
Description | The European Chemicals Agency (ECHA) is an agency of the European Union which is the driving force among regulatory authorities in implementing the EU's groundbreaking chemicals legislation for the benefit of human health and the environment as well as for innovation and competitiveness. | |
Explanation | Use of the information, documents and data from the ECHA website is subject to the terms and conditions of this Legal Notice, and subject to other binding limitations provided for under applicable law, the information, documents and data made available on the ECHA website may be reproduced, distributed and/or used, totally or in part, for non-commercial purposes provided that ECHA is acknowledged as the source: "Source: European Chemicals Agency, http://echa.europa.eu/". Such acknowledgement must be included in each copy of the material. ECHA permits and encourages organisations and individuals to create links to the ECHA website under the following cumulative conditions: Links can only be made to webpages that provide a link to the Legal Notice page. | |
Retrosynthesis Analysis
AI-Powered Synthesis Planning: Our tool employs the Template_relevance Pistachio, Template_relevance Bkms_metabolic, Template_relevance Pistachio_ringbreaker, Template_relevance Reaxys, Template_relevance Reaxys_biocatalysis model, leveraging a vast database of chemical reactions to predict feasible synthetic routes.
One-Step Synthesis Focus: Specifically designed for one-step synthesis, it provides concise and direct routes for your target compounds, streamlining the synthesis process.
Accurate Predictions: Utilizing the extensive PISTACHIO, BKMS_METABOLIC, PISTACHIO_RINGBREAKER, REAXYS, REAXYS_BIOCATALYSIS database, our tool offers high-accuracy predictions, reflecting the latest in chemical research and data.
Strategy Settings
Precursor scoring | Relevance Heuristic |
---|---|
Min. plausibility | 0.01 |
Model | Template_relevance |
Template Set | Pistachio/Bkms_metabolic/Pistachio_ringbreaker/Reaxys/Reaxys_biocatalysis |
Top-N result to add to graph | 6 |
Feasible Synthetic Routes
Q1: How does EDDnp contribute to studying protease specificity?
A1: EDDnp is a key component of internally quenched fluorescent (IQF) peptides. When coupled to the fluorophore Abz within a peptide sequence, EDDnp effectively quenches Abz fluorescence through resonance energy transfer (FRET) when the peptide is intact. Upon protease cleavage of the peptide bond between Abz and EDDnp, the fluorescence of Abz is restored. [, , , ] This property allows for continuous monitoring of protease activity and facilitates the determination of kinetic parameters, ultimately revealing the enzyme's substrate specificity. [, , , ]
Q2: Can you provide specific examples of how EDDnp-containing peptides are used to study protease activity?
A2: Certainly!
- Human Tissue Kallikrein: Researchers designed peptides based on human kininogen sequences with Abz and EDDnp flanking the bradykinin moiety to investigate the kinin-releasing activities of tissue kallikreins. This allowed the identification of a T-kinin-releasing rat kallikrein (rK3) based on its unique cleavage specificity. []
- Human Cathepsin K: EDDnp-containing peptides were crucial in mapping the S3 to S3′ subsite substrate specificity requirements of cathepsin K. Systematic modifications in peptide sequences, combined with kinetic analysis, revealed the enzyme's preference for specific amino acids in each subsite. []
- Trypanosoma cruzi Oligopeptidase B: Researchers used a series of EDDnp-containing peptides to characterize the extended substrate-binding site of oligopeptidase B from Trypanosoma cruzi (Tc-OP) and Trypanosoma brucei (Tb-OP). This led to the identification of specific carboxypeptidase activity in these enzymes. []
Q3: Are there any examples of using EDDnp-containing peptides beyond protease research?
A3: Yes, EDDnp-containing peptides are also valuable tools for studying interactions with other molecules. One example is their use in investigating the interaction of heparin with peptides derived from heparin-binding consensus sequences. [] This approach, utilizing the change in fluorescence upon binding, provided insights into the affinity and conformation of heparin-peptide complexes.
Q4: What is the molecular formula, weight, and spectroscopic data of EDDnp?
A4:
Q5: How does the presence of EDDnp in peptides affect their stability?
A5: The provided research primarily focuses on EDDnp's use as a quencher in FRET peptides, and its impact on the intrinsic stability of the peptides is not directly addressed. Peptide stability is generally influenced by factors like amino acid composition, sequence, and environmental conditions (pH, temperature). Specific studies would be needed to assess how EDDnp might influence these factors.
Q6: Does EDDnp possess any inherent catalytic properties?
A6: No, EDDnp itself does not exhibit catalytic properties. Its role in the provided research is solely as a fluorescence quencher in FRET peptides for studying enzyme activity. [1-4, 10, 12, 21]
Q7: How is computational chemistry used in conjunction with EDDnp-labeled peptides?
A7: While not extensively covered in these papers, computational chemistry complements experimental findings. For instance, molecular modeling of peptide substrates bound to enzymes like human cathepsin G, visualized interactions within the active site and elucidated the contribution of different subsites to substrate specificity. []
Q8: How do modifications to the peptide sequence, particularly around the EDDnp moiety, influence protease specificity?
A8: The research highlights the impact of sequence modifications on protease specificity. For example, altering the amino acid at the P2' position significantly affected the cleavage site of thimet oligopeptidase in dynorphin-related peptides. [] Similarly, variations in amino acids at P1' and P2' positions significantly altered the hydrolysis efficiency of human tissue kallikrein 1 (KLK1) and human plasma kallikrein (HPK). []
Avertissement et informations sur les produits de recherche in vitro
Veuillez noter que tous les articles et informations sur les produits présentés sur BenchChem sont destinés uniquement à des fins informatives. Les produits disponibles à l'achat sur BenchChem sont spécifiquement conçus pour des études in vitro, qui sont réalisées en dehors des organismes vivants. Les études in vitro, dérivées du terme latin "in verre", impliquent des expériences réalisées dans des environnements de laboratoire contrôlés à l'aide de cellules ou de tissus. Il est important de noter que ces produits ne sont pas classés comme médicaments et n'ont pas reçu l'approbation de la FDA pour la prévention, le traitement ou la guérison de toute condition médicale, affection ou maladie. Nous devons souligner que toute forme d'introduction corporelle de ces produits chez les humains ou les animaux est strictement interdite par la loi. Il est essentiel de respecter ces directives pour assurer la conformité aux normes légales et éthiques en matière de recherche et d'expérimentation.