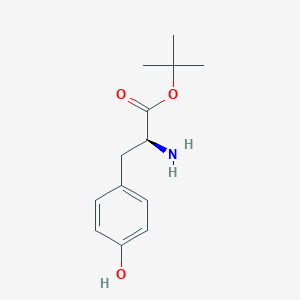
H-Tyr-OtBu
Vue d'ensemble
Description
L-Tyrosine tert-butyl ester: is a derivative of the amino acid L-Tyrosine, where the carboxyl group is protected by a tert-butyl ester. This compound is primarily used in peptide synthesis and other organic synthesis applications due to its stability and reactivity .
Applications De Recherche Scientifique
Chemistry: L-Tyrosine tert-butyl ester is widely used in peptide synthesis as a protected form of L-Tyrosine. It allows for selective deprotection and incorporation into peptide chains .
Biology: In biological research, L-Tyrosine tert-butyl ester is used to study the biosynthesis of catecholamines, such as dopamine and norepinephrine, in human keratinocytes .
Medicine: The compound is used in the development of pharmaceuticals, particularly in the synthesis of peptide-based drugs and as a precursor for various bioactive molecules .
Industry: L-Tyrosine tert-butyl ester is used as a food additive and flavor enhancer in the food industry. It improves the flavor and aroma of food products .
Mécanisme D'action
Target of Action
H-Tyr-OtBu, also known as L-Tyrosine tert-butyl ester, is a useful organic compound for research related to life sciences . .
Mode of Action
It’s often used in peptide synthesis , suggesting it may interact with proteins or enzymes in the body
Biochemical Pathways
Given its role in peptide synthesis , it may be involved in protein-related pathways. More research is needed to identify the exact pathways and their downstream effects.
Result of Action
As a compound used in peptide synthesis , it may contribute to the formation of specific proteins or peptides
Analyse Biochimique
Biochemical Properties
Tert-butyl (2S)-2-amino-3-(4-hydroxyphenyl)propanoate plays a significant role in biochemical reactions, particularly in peptide synthesis. It interacts with various enzymes and proteins involved in these processes. For instance, it is commonly used as a substrate in solid-phase peptide synthesis, where it undergoes coupling reactions facilitated by peptide bond-forming enzymes. The tert-butyl group provides protection to the amino acid, preventing unwanted side reactions during synthesis. Additionally, tert-butyl (2S)-2-amino-3-(4-hydroxyphenyl)propanoate can interact with proteases and peptidases, which can cleave the ester bond to release the free amino acid, L-tyrosine .
Cellular Effects
Tert-butyl (2S)-2-amino-3-(4-hydroxyphenyl)propanoate influences various cellular processes due to its role as a precursor to L-tyrosine. L-tyrosine is essential for protein synthesis and is involved in the production of neurotransmitters such as dopamine, norepinephrine, and epinephrine. The presence of tert-butyl (2S)-2-amino-3-(4-hydroxyphenyl)propanoate in cells can enhance the availability of L-tyrosine, thereby influencing cell signaling pathways, gene expression, and cellular metabolism. It has been observed that variations in L-tyrosine levels can affect cell growth, differentiation, and apoptosis .
Molecular Mechanism
The molecular mechanism of action of tert-butyl (2S)-2-amino-3-(4-hydroxyphenyl)propanoate involves its conversion to L-tyrosine through enzymatic cleavage of the ester bond. This conversion is typically mediated by esterases or peptidases. Once converted to L-tyrosine, it can be incorporated into proteins or serve as a precursor for the synthesis of catecholamines. The binding interactions of tert-butyl (2S)-2-amino-3-(4-hydroxyphenyl)propanoate with enzymes and proteins are crucial for its function in biochemical reactions. Additionally, it can influence gene expression by modulating the levels of L-tyrosine, which in turn affects the synthesis of proteins and neurotransmitters .
Temporal Effects in Laboratory Settings
In laboratory settings, the effects of tert-butyl (2S)-2-amino-3-(4-hydroxyphenyl)propanoate can change over time due to its stability and degradation. The compound is relatively stable under standard storage conditions, but it can undergo hydrolysis to release L-tyrosine over extended periods. This degradation can impact the long-term effects on cellular function, as the availability of L-tyrosine may fluctuate. In in vitro studies, the stability of tert-butyl (2S)-2-amino-3-(4-hydroxyphenyl)propanoate is crucial for consistent experimental outcomes .
Dosage Effects in Animal Models
The effects of tert-butyl (2S)-2-amino-3-(4-hydroxyphenyl)propanoate in animal models vary with different dosages. At lower doses, it can enhance the availability of L-tyrosine, supporting normal cellular functions and metabolic processes. At higher doses, it may lead to toxic or adverse effects due to the accumulation of L-tyrosine or its metabolites. Studies have shown that excessive levels of L-tyrosine can disrupt neurotransmitter balance and cause oxidative stress, leading to cellular damage .
Metabolic Pathways
Tert-butyl (2S)-2-amino-3-(4-hydroxyphenyl)propanoate is involved in metabolic pathways related to amino acid metabolism. Once converted to L-tyrosine, it participates in the biosynthesis of catecholamines and other important metabolites. The enzymes phenylalanine hydroxylase and tyrosine hydroxylase play key roles in these pathways, converting phenylalanine to L-tyrosine and L-tyrosine to L-DOPA, respectively. These metabolic pathways are essential for maintaining cellular homeostasis and supporting various physiological functions .
Transport and Distribution
Within cells and tissues, tert-butyl (2S)-2-amino-3-(4-hydroxyphenyl)propanoate is transported and distributed through specific transporters and binding proteins. These transporters facilitate the uptake and release of the compound, ensuring its availability for biochemical reactions. The distribution of tert-butyl (2S)-2-amino-3-(4-hydroxyphenyl)propanoate can influence its localization and accumulation within different cellular compartments, affecting its activity and function .
Subcellular Localization
The subcellular localization of tert-butyl (2S)-2-amino-3-(4-hydroxyphenyl)propanoate is influenced by targeting signals and post-translational modifications. These signals direct the compound to specific compartments or organelles, where it can exert its effects. For example, the presence of specific targeting sequences can direct tert-butyl (2S)-2-amino-3-(4-hydroxyphenyl)propanoate to the mitochondria or endoplasmic reticulum, influencing its role in cellular metabolism and protein synthesis .
Méthodes De Préparation
Synthetic Routes and Reaction Conditions:
Classical Method: The preparation of L-Tyrosine tert-butyl ester typically involves the esterification of L-Tyrosine with tert-butanol in the presence of an acid catalyst such as sulfuric acid or hydrochloric acid. The reaction is carried out under reflux conditions to ensure complete esterification.
Modern Method: A more efficient and safer method involves the use of boron trifluoride etherate adsorbed on anhydrous magnesium sulfate as a catalyst.
Industrial Production Methods: Industrial production of L-Tyrosine tert-butyl ester follows similar synthetic routes but on a larger scale. The use of continuous flow reactors and automated systems ensures consistent quality and high throughput .
Analyse Des Réactions Chimiques
Types of Reactions:
Reduction: Reduction reactions can be performed using reagents such as lithium aluminum hydride or sodium borohydride.
Common Reagents and Conditions:
Oxidation: Potassium permanganate, chromium trioxide.
Reduction: Lithium aluminum hydride, sodium borohydride.
Substitution: Various nucleophiles such as amines or alcohols.
Major Products Formed:
Oxidation: Oxidized derivatives of L-Tyrosine.
Reduction: Reduced forms of L-Tyrosine derivatives.
Substitution: Substituted L-Tyrosine derivatives with different functional groups.
Comparaison Avec Des Composés Similaires
O-tert-Butyl-L-tyrosine: Another protected form of L-Tyrosine used in peptide synthesis.
L-Phenylalanine tert-butyl ester: A protected form of L-Phenylalanine used in similar applications.
Fmoc-Lys (Boc)-OH: A protected form of L-Lysine used in peptide synthesis.
Uniqueness: L-Tyrosine tert-butyl ester is unique due to its specific protection of the carboxyl group, which allows for selective reactions in peptide synthesis. Its stability and ease of deprotection make it a valuable reagent in both research and industrial applications .
Propriétés
IUPAC Name |
tert-butyl (2S)-2-amino-3-(4-hydroxyphenyl)propanoate | |
---|---|---|
Source | PubChem | |
URL | https://pubchem.ncbi.nlm.nih.gov | |
Description | Data deposited in or computed by PubChem | |
InChI |
InChI=1S/C13H19NO3/c1-13(2,3)17-12(16)11(14)8-9-4-6-10(15)7-5-9/h4-7,11,15H,8,14H2,1-3H3/t11-/m0/s1 | |
Source | PubChem | |
URL | https://pubchem.ncbi.nlm.nih.gov | |
Description | Data deposited in or computed by PubChem | |
InChI Key |
DIGHFXIWRPMGSA-NSHDSACASA-N | |
Source | PubChem | |
URL | https://pubchem.ncbi.nlm.nih.gov | |
Description | Data deposited in or computed by PubChem | |
Canonical SMILES |
CC(C)(C)OC(=O)C(CC1=CC=C(C=C1)O)N | |
Source | PubChem | |
URL | https://pubchem.ncbi.nlm.nih.gov | |
Description | Data deposited in or computed by PubChem | |
Isomeric SMILES |
CC(C)(C)OC(=O)[C@H](CC1=CC=C(C=C1)O)N | |
Source | PubChem | |
URL | https://pubchem.ncbi.nlm.nih.gov | |
Description | Data deposited in or computed by PubChem | |
Molecular Formula |
C13H19NO3 | |
Source | PubChem | |
URL | https://pubchem.ncbi.nlm.nih.gov | |
Description | Data deposited in or computed by PubChem | |
DSSTOX Substance ID |
DTXSID301297192 | |
Record name | L-Tyrosine 1,1-dimethylethyl ester | |
Source | EPA DSSTox | |
URL | https://comptox.epa.gov/dashboard/DTXSID301297192 | |
Description | DSSTox provides a high quality public chemistry resource for supporting improved predictive toxicology. | |
Molecular Weight |
237.29 g/mol | |
Source | PubChem | |
URL | https://pubchem.ncbi.nlm.nih.gov | |
Description | Data deposited in or computed by PubChem | |
CAS No. |
16874-12-7 | |
Record name | L-Tyrosine 1,1-dimethylethyl ester | |
Source | CAS Common Chemistry | |
URL | https://commonchemistry.cas.org/detail?cas_rn=16874-12-7 | |
Description | CAS Common Chemistry is an open community resource for accessing chemical information. Nearly 500,000 chemical substances from CAS REGISTRY cover areas of community interest, including common and frequently regulated chemicals, and those relevant to high school and undergraduate chemistry classes. This chemical information, curated by our expert scientists, is provided in alignment with our mission as a division of the American Chemical Society. | |
Explanation | The data from CAS Common Chemistry is provided under a CC-BY-NC 4.0 license, unless otherwise stated. | |
Record name | L-Tyrosine 1,1-dimethylethyl ester | |
Source | EPA DSSTox | |
URL | https://comptox.epa.gov/dashboard/DTXSID301297192 | |
Description | DSSTox provides a high quality public chemistry resource for supporting improved predictive toxicology. | |
Record name | tert-butyl L-tyrosinate | |
Source | European Chemicals Agency (ECHA) | |
URL | https://echa.europa.eu/substance-information/-/substanceinfo/100.037.169 | |
Description | The European Chemicals Agency (ECHA) is an agency of the European Union which is the driving force among regulatory authorities in implementing the EU's groundbreaking chemicals legislation for the benefit of human health and the environment as well as for innovation and competitiveness. | |
Explanation | Use of the information, documents and data from the ECHA website is subject to the terms and conditions of this Legal Notice, and subject to other binding limitations provided for under applicable law, the information, documents and data made available on the ECHA website may be reproduced, distributed and/or used, totally or in part, for non-commercial purposes provided that ECHA is acknowledged as the source: "Source: European Chemicals Agency, http://echa.europa.eu/". Such acknowledgement must be included in each copy of the material. ECHA permits and encourages organisations and individuals to create links to the ECHA website under the following cumulative conditions: Links can only be made to webpages that provide a link to the Legal Notice page. | |
Retrosynthesis Analysis
AI-Powered Synthesis Planning: Our tool employs the Template_relevance Pistachio, Template_relevance Bkms_metabolic, Template_relevance Pistachio_ringbreaker, Template_relevance Reaxys, Template_relevance Reaxys_biocatalysis model, leveraging a vast database of chemical reactions to predict feasible synthetic routes.
One-Step Synthesis Focus: Specifically designed for one-step synthesis, it provides concise and direct routes for your target compounds, streamlining the synthesis process.
Accurate Predictions: Utilizing the extensive PISTACHIO, BKMS_METABOLIC, PISTACHIO_RINGBREAKER, REAXYS, REAXYS_BIOCATALYSIS database, our tool offers high-accuracy predictions, reflecting the latest in chemical research and data.
Strategy Settings
Precursor scoring | Relevance Heuristic |
---|---|
Min. plausibility | 0.01 |
Model | Template_relevance |
Template Set | Pistachio/Bkms_metabolic/Pistachio_ringbreaker/Reaxys/Reaxys_biocatalysis |
Top-N result to add to graph | 6 |
Feasible Synthetic Routes
Q1: What is L-Tyrosine tert-butyl ester (BTBE) and why is it used in biological research?
A1: L-Tyrosine tert-butyl ester (BTBE) is a chemically modified form of the amino acid tyrosine. It's primarily used as a research tool to study oxidative processes in hydrophobic environments like cell membranes and lipoproteins [, , ]. This is because BTBE, unlike natural tyrosine, can be easily incorporated into these hydrophobic environments due to its tert-butyl ester group.
Q2: How does BTBE help researchers understand tyrosine nitration and dimerization?
A2: BTBE serves as a valuable probe for studying the mechanisms of tyrosine nitration and dimerization, processes often associated with oxidative stress and various diseases [, ]. When incorporated into liposomes, BTBE's behavior upon reaction with oxidants like peroxynitrite helps scientists understand how these modifications happen to tyrosine residues within cell membranes [, ].
Q3: What have researchers learned about BTBE's interaction with peroxynitrite?
A3: Studies using BTBE incorporated into liposomes have revealed that peroxynitrite can cause both nitration and dimerization of BTBE, much like it does with tyrosine in aqueous solutions []. This finding suggests that peroxynitrite can penetrate hydrophobic environments and modify tyrosine residues within them [, ].
Q4: How does the presence of carbon dioxide (CO2) affect BTBE nitration by peroxynitrite?
A4: Interestingly, the presence of CO2, a common molecule in biological systems, has been shown to decrease BTBE nitration within liposomes, unlike its effect on tyrosine in aqueous solutions [, ]. This suggests that the carbonate radical (CO3•-), formed from the reaction of CO2 with peroxynitrite, might have limited ability to penetrate the hydrophobic environment where BTBE is located [, ].
Q5: What is the role of lipid peroxidation in BTBE oxidation?
A5: Research suggests that lipid peroxidation, a process where free radicals attack lipids, plays a significant role in BTBE oxidation in membranes []. Lipid peroxyl radicals (LOO•), generated during lipid peroxidation, have been shown to directly react with BTBE, leading to its oxidation []. This highlights the interconnectedness of lipid and protein oxidation in biological systems.
Q6: How does BTBE compare to its phenylalanine analogue (BPBE) in terms of reacting with lipid peroxyl radicals?
A6: Unlike BTBE, its phenylalanine analogue (BPBE) does not significantly inhibit oxidant-stimulated oxygen consumption in liposomes []. This difference implies that the phenol moiety present in BTBE, but absent in BPBE, is crucial for its reactivity with lipid peroxyl radicals [].
Avertissement et informations sur les produits de recherche in vitro
Veuillez noter que tous les articles et informations sur les produits présentés sur BenchChem sont destinés uniquement à des fins informatives. Les produits disponibles à l'achat sur BenchChem sont spécifiquement conçus pour des études in vitro, qui sont réalisées en dehors des organismes vivants. Les études in vitro, dérivées du terme latin "in verre", impliquent des expériences réalisées dans des environnements de laboratoire contrôlés à l'aide de cellules ou de tissus. Il est important de noter que ces produits ne sont pas classés comme médicaments et n'ont pas reçu l'approbation de la FDA pour la prévention, le traitement ou la guérison de toute condition médicale, affection ou maladie. Nous devons souligner que toute forme d'introduction corporelle de ces produits chez les humains ou les animaux est strictement interdite par la loi. Il est essentiel de respecter ces directives pour assurer la conformité aux normes légales et éthiques en matière de recherche et d'expérimentation.