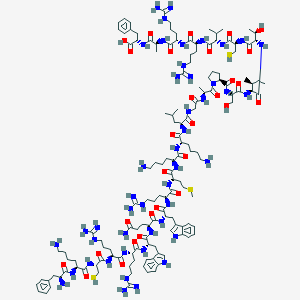
Lactoferricin B
- Cliquez sur DEMANDE RAPIDE pour recevoir un devis de notre équipe d'experts.
- Avec des produits de qualité à un prix COMPÉTITIF, vous pouvez vous concentrer davantage sur votre recherche.
Vue d'ensemble
Description
Lactoferricin B (LfcinB), a 25-residue cationic peptide derived from bovine lactoferrin, exhibits broad-spectrum antimicrobial and anticancer activities. Its mechanism involves rapid binding to microbial or cancer cell membranes via electrostatic interactions, followed by disruption of membrane integrity through hydrophobic residues (Phe1, Trp6, Trp8, etc.) . Structurally, LfcinB adopts a distorted antiparallel β-sheet conformation in solution, creating an amphipathic surface critical for membrane permeabilization . Beyond direct membrane disruption, LfcinB inhibits bacterial topoisomerases (e.g., gyrase and Topo IV) and disrupts cancer cell mitochondrial functions, enhancing reactive oxygen species (ROS) generation . Notably, it demonstrates selective toxicity toward leukemic cells (EC50 ratios >2.0 vs. healthy cells), attributed to altered phosphatidylserine exposure on cancer membranes .
Méthodes De Préparation
Synthetic Routes and Reaction Conditions
Lactoferricin B can be synthesized through recombinant DNA technology. The gene encoding this compound is inserted into an expression vector, which is then introduced into a host organism, such as Escherichia coli. The host organism produces the peptide, which is subsequently purified . The peptide can also be chemically synthesized using solid-phase peptide synthesis, which involves the sequential addition of amino acids to a growing peptide chain anchored to a solid support .
Industrial Production Methods
Industrial production of this compound typically involves large-scale fermentation processes using genetically modified microorganisms. The fermentation broth is then subjected to various purification steps, including chromatography and ultrafiltration, to isolate and purify the peptide .
Analyse Des Réactions Chimiques
Interaction with Bacterial Membranes
Lfcin B disrupts membrane integrity through electrostatic and hydrophobic interactions. In Escherichia coli, Lfcin B binds to anionic phospholipids like phosphatidylglycerol, increasing the outer monolayer area of lipid bilayers and inducing rapid leakage of cytoplasmic contents. Studies using giant unilamellar vesicles (GUVs) revealed:
- Local rupture mechanism : Lfcin B causes stochastic membrane damage, leading to rapid efflux of small molecules (e.g., calcein) without forming stable pores .
- Charge dependency : Membrane disruption efficacy correlates with surface charge density, with higher activity in negatively charged membranes .
Table 1: Membrane Disruption Parameters of Lfcin B
Interference with Two-Component Systems (TCS)
Lfcin B directly inhibits TCS phosphorylation in E. coli:
- Targets : Binds response regulators BasR and CreB, blocking their phosphorylation by sensor kinases BasS and CreC .
- Functional impact : Reduces bacterial tolerance to ferric ions and nutrient deprivation .
Table 3: Affected Two-Component System Components
Component | Role in E. coli | Effect of Lfcin B | Source |
---|---|---|---|
BasR/BasS (PmrAB) | Regulates iron homeostasis | Inhibits phosphorylation | |
CreB/CreC | Carbon catabolite repression | Blocks DNA-binding ability |
Interaction with Metabolic Enzymes
Proteome-wide screens identified intracellular targets linked to central metabolism:
- Key enzymes : Phosphoenolpyruvate carboxylase (PPC), isocitrate dehydrogenase (Icd), and pyruvate kinase (PykF) .
- Metabolic dysregulation : Lfcin B exposure increases pyruvate accumulation by 2.5-fold in E. coli, disrupting the TCA cycle .
Table 4: Metabolic Targets of Lfcin B
Enzyme | Pathway | Functional Consequence | Source |
---|---|---|---|
Phosphoenolpyruvate carboxylase | Pyruvate metabolism | Pyruvate accumulation | |
Isocitrate dehydrogenase | TCA cycle | Reduced α-ketoglutarate production |
Structural Basis of Activity
NMR studies reveal conformational plasticity:
- Aqueous solution : Disordered structure with nascent helices .
- Membrane-mimetic solvents : Adopts amphipathic β-sheet or helical conformations, enhancing lipid interaction .
Table 5: Structural Features of Lfcin B
Solvent Environment | Secondary Structure | Key Residues Involved | Source |
---|---|---|---|
Aqueous | Disordered/coiled | Gln14–Lys29 (nascent helix) | |
DOPG/DOPC membranes | β-sheet or helical motifs | Trp6, Arg12, Phe17 |
Lfcin B’s bactericidal activity arises from a combination of membrane disruption, macromolecular synthesis inhibition, and metabolic interference. Its ability to target both structural and regulatory components underscores broad-spectrum efficacy, making it a model for designing peptide-based antimicrobials.
Applications De Recherche Scientifique
Lactoferricin B has numerous scientific research applications across various fields:
Mécanisme D'action
Lactoferricin B exerts its effects primarily through interactions with microbial membranes. The peptide’s amphipathic structure allows it to insert into and disrupt the lipid bilayer of microbial cells, leading to cell lysis and death . Additionally, this compound can bind to and neutralize lipopolysaccharides on the surface of Gram-negative bacteria, further enhancing its antimicrobial activity . The peptide also exhibits immunomodulatory effects by interacting with immune cells and modulating their activity .
Comparaison Avec Des Composés Similaires
Comparative Analysis with Similar Compounds
Antimicrobial Activity and Selectivity
Table 1: Antimicrobial Peptide Comparison
Notes:
- LfcinB vs. Magainin 2 : LfcinB exhibits lower MICs against S. aureus (8 vs. 16–32 μg/mL) and broader antifungal activity .
- D-Amino Acid Peptides (e.g., NY15): While D-isomers like NY15 show superior MICs due to protease resistance, LfcinB’s D-form retains comparable activity without stereo-specific receptor dependency .
- Synergy: LfcinB enhances fluoroquinolones (e.g., ciprofloxacin) and azoles (e.g., fluconazole) by disrupting microbial membranes, improving drug uptake .
Anticancer Activity and Selectivity
Table 2: Anticancer Peptide Comparison
Key Findings :
- Leukemic Selectivity : LfcinB’s EC50 ratio of >2.0 for leukemia vs. healthy cells surpasses defensins (1.5–2.0) and LFchimera (1.8) due to preferential binding to anionic phosphatidylserine on cancer membranes .
- Mitochondrial Targeting : Unlike melimine and defensins, LfcinB disrupts both cytoplasmic and mitochondrial membranes, amplifying ROS-mediated apoptosis .
Structural and Functional Divergence
- Amphipathicity : LfcinB’s β-sheet structure contrasts with α-helical peptides like LL-37 and Cecropin A, enabling distinct membrane interaction modes .
- Enzymatic Targets : LfcinB uniquely inhibits bacterial topoisomerases (IC50 = 0.5–2 μM for gyrase) and binds eukaryotic transcription-related proteins (e.g., RNA polymerase), unlike Cecropin A or Magainin 2 .
Activité Biologique
Lactoferricin B (LfcinB) is a cationic antimicrobial peptide derived from bovine lactoferrin, known for its diverse biological activities, including antimicrobial, anticancer, and immunomodulatory effects. This article reviews the current understanding of LfcinB's biological activity, supported by recent research findings and case studies.
Antimicrobial Activity
Mechanisms of Action
LfcinB exhibits a multifaceted mechanism of action against various pathogens, primarily through its interaction with microbial membranes and intracellular targets. Key studies have demonstrated that LfcinB can:
- Inhibit Bacterial Growth : LfcinB disrupts the integrity of bacterial membranes, leading to cell lysis in susceptible strains. It has been shown to affect the synthesis of macromolecules such as DNA, RNA, and proteins in bacteria like Escherichia coli and Bacillus subtilis .
- Target Intracellular Pathways : Research indicates that LfcinB binds to specific intracellular targets, including response regulators in two-component systems (BasR and CreB), inhibiting their phosphorylation and affecting bacterial signaling pathways .
Table 1: Summary of Antimicrobial Activity of this compound
Anticancer Activity
Induction of Apoptosis
LfcinB has shown promising results in inducing apoptosis in various cancer cell lines without affecting normal cells. For instance, studies have reported that LfcinB selectively induces apoptosis in human gastric cancer cells (AGS) by inhibiting autophagy at late stages . Additionally, it has been effective against leukemia cells, enhancing survival rates in animal models bearing tumor xenografts .
Case Study: Gastric Cancer Cells
In a study examining the effects of LfcinB on gastric cancer cell line AGS:
- Findings : LfcinB induced apoptosis through a mechanism involving caspase-independent pathways and modulation of autophagy.
- Implications : These findings suggest potential therapeutic applications for LfcinB in treating gastric cancer .
Immunomodulatory Effects
LfcinB also exhibits immunomodulatory properties, enhancing the immune response against infections. It has been shown to stimulate the production of cytokines and chemokines, contributing to the host's defense mechanisms against pathogens .
Synergistic Effects with Antibiotics
Recent studies have explored the synergistic effects of LfcinB when combined with conventional antibiotics. This combination has demonstrated enhanced efficacy against resistant strains of bacteria, such as methicillin-resistant Staphylococcus aureus (MRSA) . The dual functionality of these conjugates is particularly beneficial in cancer treatment where patients are at higher risk for infections.
Q & A
Basic Research Questions
Q. What experimental models are commonly used to study the antimicrobial mechanisms of Lactoferricin B?
- Methodological Answer : Researchers employ in vitro assays such as fluorimetry using the fluorescent probe 1-N-phenylnaphthylamine (NPN) to measure outer membrane permeabilization in Gram-negative bacteria . Minimum Inhibitory Concentration (MIC) assays are standard for evaluating antimicrobial efficacy against diverse bacterial strains, with protocols standardized by CLSI guidelines. Structural studies often utilize nuclear magnetic resonance (NMR) or circular dichroism (CD) spectroscopy in membrane-mimetic environments (e.g., sodium dodecyl sulfate micelles) to elucidate peptide-lipid interactions .
Table 1 : Common Experimental Models for this compound Studies
Q. How is this compound sourced and purified for academic research?
- Methodological Answer : this compound is typically derived from enzymatic cleavage of lactoferrin (e.g., pepsin digestion) or synthesized via solid-phase peptide synthesis (SPPS). Purification involves reversed-phase high-performance liquid chromatography (RP-HPLC) with C18 columns, followed by mass spectrometry (MS) for validation of molecular weight and purity . Recombinant expression in E. coli systems is also used, requiring codon optimization and inclusion body refolding protocols .
Advanced Research Questions
Q. What methodologies are used to analyze the relationship between this compound’s structural motifs and its antimicrobial activity?
- Methodological Answer : Structure-activity relationship (SAR) studies combine mutagenesis with biophysical assays. For example, truncation or substitution of arginine/tryptophan residues (critical for membrane interaction) is assessed via MIC assays and fluorescence microscopy. Synchrotron radiation small-angle X-ray scattering (SAXS) can map peptide-lipid bilayer interactions in real time .
Q. How can researchers address conflicting data on this compound’s cytotoxicity in eukaryotic cells?
- Methodological Answer : Contradictions often arise from variations in cell lines, peptide concentrations, and exposure times. Standardized cytotoxicity assays (e.g., MTT or LDH release) should be paired with hemolysis tests using erythrocytes. Dose-response curves and time-lapse imaging can differentiate between selective antimicrobial activity and non-specific cytotoxicity .
Q. What strategies improve the stability and bioavailability of this compound in in vivo models?
- Methodological Answer : Cyclization via disulfide bonds or PEGylation reduces proteolytic degradation. Encapsulation in liposomes or nanoparticles enhances tissue targeting. Pharmacokinetic studies using radiolabeled peptides (e.g., tritium) or HPLC-MS quantify bioavailability in serum .
Q. Data Analysis & Experimental Design
Q. How should researchers design experiments to resolve contradictions in reported MIC values across studies?
- Methodological Answer : Controlled variables include bacterial growth phase (log vs. stationary), culture media composition, and peptide solubilization buffers. Statistical frameworks like ANOVA with post-hoc tests (e.g., Tukey’s HSD) account for inter-experimental variability. Meta-analyses of published data using PRISMA guidelines can identify confounding factors .
Q. What are best practices for validating this compound’s immunomodulatory effects in complex biological systems?
- Methodological Answer : Use primary immune cells (e.g., macrophages) and cytokine profiling (ELISA or multiplex assays) to measure inflammatory responses. In vivo models (e.g., murine infection) require ethical approval and strict adherence to ARRIVE guidelines for experimental rigor. Flow cytometry tracks immune cell activation markers (e.g., CD86/CD206) .
Propriétés
Numéro CAS |
146897-68-9 |
---|---|
Formule moléculaire |
C141H224N46O29S3 |
Poids moléculaire |
3123.8 g/mol |
Nom IUPAC |
(2S)-2-[[(2S)-2-[[(2S)-2-[[(2S)-2-[[(2S)-2-[[(3S,9S,12S,15S,18S,21S,24S,27S,30S,33S,36S,39R,44R,47S,50S,53S,56S)-39-[[(2S)-6-amino-2-[[(2S)-2-amino-3-phenylpropanoyl]amino]hexanoyl]amino]-12,15-bis(4-aminobutyl)-27-(3-amino-3-oxopropyl)-50-[(2S)-butan-2-yl]-21,33,36-tris(3-carbamimidamidopropyl)-47-[(1R)-1-hydroxyethyl]-53-(hydroxymethyl)-24,30-bis(1H-indol-3-ylmethyl)-3-methyl-9-(2-methylpropyl)-18-(2-methylsulfanylethyl)-2,5,8,11,14,17,20,23,26,29,32,35,38,46,49,52,55-heptadecaoxo-41,42-dithia-1,4,7,10,13,16,19,22,25,28,31,34,37,45,48,51,54-heptadecazabicyclo[54.3.0]nonapentacontane-44-carbonyl]amino]-3-methylbutanoyl]amino]-5-carbamimidamidopentanoyl]amino]-5-carbamimidamidopentanoyl]amino]propanoyl]amino]-3-phenylpropanoic acid |
InChI |
InChI=1S/C141H224N46O29S3/c1-11-77(6)111-133(212)186-112(80(9)189)134(213)183-106(130(209)184-110(76(4)5)132(211)176-97(49-32-61-161-141(155)156)120(199)170-93(45-28-57-157-137(147)148)116(195)166-78(7)113(192)180-103(136(215)216)66-82-36-16-13-17-37-82)74-219-218-73-105(182-123(202)90(42-22-25-54-142)167-114(193)87(145)65-81-34-14-12-15-35-81)129(208)173-95(47-30-59-159-139(151)152)118(197)171-96(48-31-60-160-140(153)154)122(201)178-102(68-84-70-163-89-41-21-19-39-86(84)89)127(206)174-98(51-52-108(146)190)124(203)179-101(67-83-69-162-88-40-20-18-38-85(83)88)126(205)172-94(46-29-58-158-138(149)150)119(198)175-99(53-63-217-10)125(204)169-91(43-23-26-55-143)117(196)168-92(44-24-27-56-144)121(200)177-100(64-75(2)3)115(194)164-71-109(191)165-79(8)135(214)187-62-33-50-107(187)131(210)181-104(72-188)128(207)185-111/h12-21,34-41,69-70,75-80,87,90-107,110-112,162-163,188-189H,11,22-33,42-68,71-74,142-145H2,1-10H3,(H2,146,190)(H,164,194)(H,165,191)(H,166,195)(H,167,193)(H,168,196)(H,169,204)(H,170,199)(H,171,197)(H,172,205)(H,173,208)(H,174,206)(H,175,198)(H,176,211)(H,177,200)(H,178,201)(H,179,203)(H,180,192)(H,181,210)(H,182,202)(H,183,213)(H,184,209)(H,185,207)(H,186,212)(H,215,216)(H4,147,148,157)(H4,149,150,158)(H4,151,152,159)(H4,153,154,160)(H4,155,156,161)/t77-,78-,79-,80+,87-,90-,91-,92-,93-,94-,95-,96-,97-,98-,99-,100-,101-,102-,103-,104-,105-,106-,107-,110-,111-,112-/m0/s1 |
Clé InChI |
PHEGMCHJJYMADA-IKGCZBKSSA-N |
SMILES |
CCC(C)C(C(=O)NC(C(C)O)C(=O)NC(CS)C(=O)NC(C(C)C)C(=O)NC(CCCNC(=N)N)C(=O)NC(CCCNC(=N)N)C(=O)NC(C)C(=O)NC(CC1=CC=CC=C1)C(=O)O)NC(=O)C(CO)NC(=O)C2CCCN2C(=O)C(C)NC(=O)CNC(=O)C(CC(C)C)NC(=O)C(CCCCN)NC(=O)C(CCCCN)NC(=O)C(CCSC)NC(=O)C(CCCNC(=N)N)NC(=O)C(CC3=CNC4=CC=CC=C43)NC(=O)C(CCC(=O)N)NC(=O)C(CC5=CNC6=CC=CC=C65)NC(=O)C(CCCNC(=N)N)NC(=O)C(CCCNC(=N)N)NC(=O)C(CS)NC(=O)C(CCCCN)NC(=O)C(CC7=CC=CC=C7)N |
SMILES isomérique |
CC[C@H](C)[C@H]1C(=O)N[C@H](C(=O)N[C@@H](CSSC[C@@H](C(=O)N[C@H](C(=O)N[C@H](C(=O)N[C@H](C(=O)N[C@H](C(=O)N[C@H](C(=O)N[C@H](C(=O)N[C@H](C(=O)N[C@H](C(=O)N[C@H](C(=O)N[C@H](C(=O)NCC(=O)N[C@H](C(=O)N2CCC[C@H]2C(=O)N[C@H](C(=O)N1)CO)C)CC(C)C)CCCCN)CCCCN)CCSC)CCCNC(=N)N)CC3=CNC4=CC=CC=C43)CCC(=O)N)CC5=CNC6=CC=CC=C65)CCCNC(=N)N)CCCNC(=N)N)NC(=O)[C@H](CCCCN)NC(=O)[C@H](CC7=CC=CC=C7)N)C(=O)N[C@@H](C(C)C)C(=O)N[C@@H](CCCNC(=N)N)C(=O)N[C@@H](CCCNC(=N)N)C(=O)N[C@@H](C)C(=O)N[C@@H](CC8=CC=CC=C8)C(=O)O)[C@@H](C)O |
SMILES canonique |
CCC(C)C1C(=O)NC(C(=O)NC(CSSCC(C(=O)NC(C(=O)NC(C(=O)NC(C(=O)NC(C(=O)NC(C(=O)NC(C(=O)NC(C(=O)NC(C(=O)NC(C(=O)NC(C(=O)NCC(=O)NC(C(=O)N2CCCC2C(=O)NC(C(=O)N1)CO)C)CC(C)C)CCCCN)CCCCN)CCSC)CCCNC(=N)N)CC3=CNC4=CC=CC=C43)CCC(=O)N)CC5=CNC6=CC=CC=C65)CCCNC(=N)N)CCCNC(=N)N)NC(=O)C(CCCCN)NC(=O)C(CC7=CC=CC=C7)N)C(=O)NC(C(C)C)C(=O)NC(CCCNC(=N)N)C(=O)NC(CCCNC(=N)N)C(=O)NC(C)C(=O)NC(CC8=CC=CC=C8)C(=O)O)C(C)O |
Séquence |
One Letter Code: FKCRRWQWRMKKLGAPSITCVRRAF (Disulfide bridge: Cys3-Cys20) |
Synonymes |
lactoferricin lactoferricin B |
Origine du produit |
United States |
Avertissement et informations sur les produits de recherche in vitro
Veuillez noter que tous les articles et informations sur les produits présentés sur BenchChem sont destinés uniquement à des fins informatives. Les produits disponibles à l'achat sur BenchChem sont spécifiquement conçus pour des études in vitro, qui sont réalisées en dehors des organismes vivants. Les études in vitro, dérivées du terme latin "in verre", impliquent des expériences réalisées dans des environnements de laboratoire contrôlés à l'aide de cellules ou de tissus. Il est important de noter que ces produits ne sont pas classés comme médicaments et n'ont pas reçu l'approbation de la FDA pour la prévention, le traitement ou la guérison de toute condition médicale, affection ou maladie. Nous devons souligner que toute forme d'introduction corporelle de ces produits chez les humains ou les animaux est strictement interdite par la loi. Il est essentiel de respecter ces directives pour assurer la conformité aux normes légales et éthiques en matière de recherche et d'expérimentation.