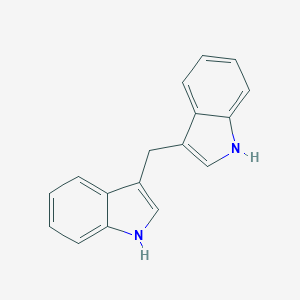
3,3'-Diindolylmethane
Vue d'ensemble
Description
3,3'-Diindolylmethane (DIM) is a naturally occurring compound found in cruciferous vegetables such as broccoli, cabbage, and cauliflower. It is a derivative of indole-3-carbinol (I3C), which is produced when these vegetables are cooked or digested. DIM has been studied extensively for its potential health benefits, including its ability to modulate the activity of the immune system, regulate cell growth, and act as an antioxidant. DIM has also been studied for its potential role in the prevention and treatment of cancer, and for its potential to reduce inflammation and treat autoimmune disorders.
Applications De Recherche Scientifique
Cancer Treatment
DIM has been found to have potent anti-cancer properties . It functions as an antitumor agent and can directly stimulate apoptosis at relatively high concentrations . It also sensitizes TRAIL-induced apoptosis in human cancer cells .
Treatment of Gastrointestinal Cancer
Studies have shown that DIM is effective in the attenuation of gastrointestinal cancers . It acts upon several cellular and molecular processes in gastrointestinal cancer cells, including apoptosis, autophagy, invasion, cell cycle regulation, metastasis, angiogenesis, and endoplasmic reticulum (ER) stress .
Treatment of Triple Negative Breast Cancer (TNBC)
DIM has been used in the treatment of TNBC . A novel approach of combinatorial treatment of DOX with dietary indole 3,3′-diindolylmethane (DIM) targets CSC mediated EMT induction in TNBC .
Treatment of Cervical Intraepithelial Neoplasia (CIN)
In a study of 78 women with CIN, using DIM intravaginally, 100% of patients on 200 mg DIM and 90.5% of women on 100 mg DIM had complete regression of CIN after 180 days .
Treatment of Infectious Diseases
DIM is currently under investigation for the prevention and treatment of infectious diseases , including Covid-19, due to its potent immune modulating and anti-inflammatory properties .
Immune Deficiency Conditions
DIM is also being investigated for its potential in treating immune deficiency conditions due to its immune modulating properties .
Mécanisme D'action
Target of Action
3,3’-Diindolylmethane (DIM) is a bioactive compound found in cruciferous vegetables . It has been shown to interact with several targets, including the aryl hydrocarbon receptor (AhR) , histone deacetylase (HDAC) , and cannabinoid receptors (CB1 and CB2) . These targets play crucial roles in cellular processes such as cell cycle regulation, apoptosis, and inflammation .
Mode of Action
DIM interacts with its targets to induce various cellular changes. For instance, it enhances the binding of RASSF1 to the Mst1/2-LATS1-Mob1 complex , stimulating the Hippo signaling pathway and supporting YAP phosphorylation , which hinders cell proliferation . Additionally, DIM acts as a histone deacetylase inhibitor , specifically against HDAC1, HDAC2, and HDAC3 . This action can lead to changes in gene expression, further influencing cellular processes.
Biochemical Pathways
DIM affects several biochemical pathways. It has been shown to modulate the HGF/c-Met/Akt and p38 cell signaling pathways . These pathways are involved in cell cycle progression, apoptosis, and cell proliferation . By influencing these pathways, DIM can alter the downstream effects, such as cell growth and survival.
Pharmacokinetics
The pharmacokinetics of DIM have been studied in healthy subjects. After intravenous administration, the plasma concentration of DIM declined quickly . A single 100 mg dose resulted in a mean maximum plasma concentration (Cmax) of 32 ng/mL and a mean area under the curve (AUC) of 128 hng/mL. A single 200 mg dose produced a mean Cmax of 104 ng/mL and a mean AUC of 553 hng/mL . These findings suggest that DIM is well tolerated at single doses of up to 200 mg .
Result of Action
The action of DIM results in several molecular and cellular effects. It has been shown to reduce oxidative stress, impede DNA synthesis, influence target cells’ activation, proliferation, and apoptosis, and inhibit proinflammatory cytokines and chemokines . These actions contribute to DIM’s potential as a chemopreventive and therapeutic agent for various cancers .
Action Environment
The action of DIM can be influenced by environmental factors. For instance, the dietary intake of cruciferous vegetables, which are the primary source of DIM, can affect the bioavailability of this compound . Furthermore, the efficacy of DIM can be enhanced when used in combination with other drugs or therapeutic chemicals . This suggests that the action, efficacy, and stability of DIM can be modulated by the environment and the presence of other compounds.
Propriétés
IUPAC Name |
3-(1H-indol-3-ylmethyl)-1H-indole | |
---|---|---|
Source | PubChem | |
URL | https://pubchem.ncbi.nlm.nih.gov | |
Description | Data deposited in or computed by PubChem | |
InChI |
InChI=1S/C17H14N2/c1-3-7-16-14(5-1)12(10-18-16)9-13-11-19-17-8-4-2-6-15(13)17/h1-8,10-11,18-19H,9H2 | |
Source | PubChem | |
URL | https://pubchem.ncbi.nlm.nih.gov | |
Description | Data deposited in or computed by PubChem | |
InChI Key |
VFTRKSBEFQDZKX-UHFFFAOYSA-N | |
Source | PubChem | |
URL | https://pubchem.ncbi.nlm.nih.gov | |
Description | Data deposited in or computed by PubChem | |
Canonical SMILES |
C1=CC=C2C(=C1)C(=CN2)CC3=CNC4=CC=CC=C43 | |
Source | PubChem | |
URL | https://pubchem.ncbi.nlm.nih.gov | |
Description | Data deposited in or computed by PubChem | |
Molecular Formula |
C17H14N2 | |
Source | PubChem | |
URL | https://pubchem.ncbi.nlm.nih.gov | |
Description | Data deposited in or computed by PubChem | |
DSSTOX Substance ID |
DTXSID8037047 | |
Record name | 3,3,-Diindolylmethane | |
Source | EPA DSSTox | |
URL | https://comptox.epa.gov/dashboard/DTXSID8037047 | |
Description | DSSTox provides a high quality public chemistry resource for supporting improved predictive toxicology. | |
Molecular Weight |
246.31 g/mol | |
Source | PubChem | |
URL | https://pubchem.ncbi.nlm.nih.gov | |
Description | Data deposited in or computed by PubChem | |
Product Name |
3,3'-Diindolylmethane | |
CAS RN |
1968-05-4 | |
Record name | 3,3′-Diindolylmethane | |
Source | CAS Common Chemistry | |
URL | https://commonchemistry.cas.org/detail?cas_rn=1968-05-4 | |
Description | CAS Common Chemistry is an open community resource for accessing chemical information. Nearly 500,000 chemical substances from CAS REGISTRY cover areas of community interest, including common and frequently regulated chemicals, and those relevant to high school and undergraduate chemistry classes. This chemical information, curated by our expert scientists, is provided in alignment with our mission as a division of the American Chemical Society. | |
Explanation | The data from CAS Common Chemistry is provided under a CC-BY-NC 4.0 license, unless otherwise stated. | |
Record name | 3,3'-Diindolylmethane | |
Source | ChemIDplus | |
URL | https://pubchem.ncbi.nlm.nih.gov/substance/?source=chemidplus&sourceid=0001968054 | |
Description | ChemIDplus is a free, web search system that provides access to the structure and nomenclature authority files used for the identification of chemical substances cited in National Library of Medicine (NLM) databases, including the TOXNET system. | |
Record name | 3,3'-diindolylmethane | |
Source | DrugBank | |
URL | https://www.drugbank.ca/drugs/DB11875 | |
Description | The DrugBank database is a unique bioinformatics and cheminformatics resource that combines detailed drug (i.e. chemical, pharmacological and pharmaceutical) data with comprehensive drug target (i.e. sequence, structure, and pathway) information. | |
Explanation | Creative Common's Attribution-NonCommercial 4.0 International License (http://creativecommons.org/licenses/by-nc/4.0/legalcode) | |
Record name | 3,3,-Diindolylmethane | |
Source | EPA DSSTox | |
URL | https://comptox.epa.gov/dashboard/DTXSID8037047 | |
Description | DSSTox provides a high quality public chemistry resource for supporting improved predictive toxicology. | |
Record name | 1H-Indole, 3,3'-methylenebis | |
Source | European Chemicals Agency (ECHA) | |
URL | https://echa.europa.eu/substance-information/-/substanceinfo/100.124.716 | |
Description | The European Chemicals Agency (ECHA) is an agency of the European Union which is the driving force among regulatory authorities in implementing the EU's groundbreaking chemicals legislation for the benefit of human health and the environment as well as for innovation and competitiveness. | |
Explanation | Use of the information, documents and data from the ECHA website is subject to the terms and conditions of this Legal Notice, and subject to other binding limitations provided for under applicable law, the information, documents and data made available on the ECHA website may be reproduced, distributed and/or used, totally or in part, for non-commercial purposes provided that ECHA is acknowledged as the source: "Source: European Chemicals Agency, http://echa.europa.eu/". Such acknowledgement must be included in each copy of the material. ECHA permits and encourages organisations and individuals to create links to the ECHA website under the following cumulative conditions: Links can only be made to webpages that provide a link to the Legal Notice page. | |
Record name | 3,3'-DIINDOLYLMETHANE | |
Source | FDA Global Substance Registration System (GSRS) | |
URL | https://gsrs.ncats.nih.gov/ginas/app/beta/substances/SSZ9HQT61Z | |
Description | The FDA Global Substance Registration System (GSRS) enables the efficient and accurate exchange of information on what substances are in regulated products. Instead of relying on names, which vary across regulatory domains, countries, and regions, the GSRS knowledge base makes it possible for substances to be defined by standardized, scientific descriptions. | |
Explanation | Unless otherwise noted, the contents of the FDA website (www.fda.gov), both text and graphics, are not copyrighted. They are in the public domain and may be republished, reprinted and otherwise used freely by anyone without the need to obtain permission from FDA. Credit to the U.S. Food and Drug Administration as the source is appreciated but not required. | |
Synthesis routes and methods
Procedure details
Retrosynthesis Analysis
AI-Powered Synthesis Planning: Our tool employs the Template_relevance Pistachio, Template_relevance Bkms_metabolic, Template_relevance Pistachio_ringbreaker, Template_relevance Reaxys, Template_relevance Reaxys_biocatalysis model, leveraging a vast database of chemical reactions to predict feasible synthetic routes.
One-Step Synthesis Focus: Specifically designed for one-step synthesis, it provides concise and direct routes for your target compounds, streamlining the synthesis process.
Accurate Predictions: Utilizing the extensive PISTACHIO, BKMS_METABOLIC, PISTACHIO_RINGBREAKER, REAXYS, REAXYS_BIOCATALYSIS database, our tool offers high-accuracy predictions, reflecting the latest in chemical research and data.
Strategy Settings
Precursor scoring | Relevance Heuristic |
---|---|
Min. plausibility | 0.01 |
Model | Template_relevance |
Template Set | Pistachio/Bkms_metabolic/Pistachio_ringbreaker/Reaxys/Reaxys_biocatalysis |
Top-N result to add to graph | 6 |
Feasible Synthetic Routes
Q & A
Q1: What is the primary mechanism of action of 3,3'-diindolylmethane (DIM) in cancer cells?
A1: DIM exhibits its anticancer activity through multiple mechanisms, making it a multi-target agent. One key mechanism involves the inhibition of various signaling pathways implicated in cancer cell growth and survival. For instance, DIM inhibits vascular endothelial growth factor (VEGF)-induced cell proliferation and DNA synthesis in human umbilical vascular endothelial cells (HUVECs) by reducing VEGF-induced extracellular signal-regulated kinase (ERK1/2) phosphorylation. [] DIM also displays a potent cytostatic effect on human endometrial cancer cells by inducing the expression of transforming growth factor-alpha (TGF-alpha) through an estrogen receptor (ER)-mediated pathway. []
Q2: How does DIM affect the cell cycle in cancer cells?
A2: DIM induces cell cycle arrest primarily at the G1 phase in various cancer cell lines, including breast, esophageal squamous cell carcinoma, and colorectal cancer cells. [, , ] This arrest is associated with decreased activities of cyclin-dependent kinases (CDKs) like CDK2, CDK4, and CDK6, as well as upregulation of CDK inhibitors like p21(WAF1/CIP1) and p27. [, ]
Q3: Does DIM induce apoptosis in cancer cells? If so, what pathways are involved?
A3: Yes, DIM demonstrates apoptosis-inducing effects in several human cancer cell lines. Studies show that DIM can induce apoptosis through both p53-dependent and p53-independent pathways. [] For example, in human cholangiocarcinoma cells, DIM enhances Fas-mediated apoptosis by inhibiting AKT signaling and FLICE-like-inhibitory-protein (FLIP). [] Additionally, DIM treatment in breast cancer cells leads to increased mitochondrial reactive oxygen species (ROS) production, which activates stress-activated pathways like p38 and c-Jun NH(2)-terminal kinase (JNK), ultimately contributing to apoptosis. []
Q4: Does DIM impact the immune system?
A4: Research suggests that DIM may modulate the immune system. For example, in the K14-HPV16 transgenic mouse model for cervical cancer, DIM administration significantly increased serum interferon-gamma (IFN-γ) levels. [] Additionally, DIM was found to inhibit the differentiation of CD103+ dendritic cells from mouse bone marrow cells, which play a role in regulating immune tolerance. []
Q5: What are the effects of DIM on angiogenesis?
A5: DIM exhibits anti-angiogenic properties by inhibiting key pro-angiogenic factors. One study revealed that DIM significantly reduced VEGF expression in human retinal pigment epithelial cells under hypoxic conditions by suppressing mitochondrial ROS production and attenuating the activation of HIF-1α and p38 MAPK/NF-κB. [] Additionally, in a rodent Matrigel plug model, DIM effectively suppressed VEGF-induced neovascularization, demonstrating its anti-angiogenic potential in vivo. []
Q6: Has DIM shown efficacy in preclinical animal models of cancer?
A6: Yes, DIM has demonstrated promising anticancer effects in various preclinical animal models. For instance, in a study using the 4T1 murine mammary carcinoma model, oral administration of DIM significantly inhibited lung metastasis. [] This effect was accompanied by a reduction in serum levels of matrix metalloproteinases (MMP)-2 and MMP-9, tissue inhibitor of metalloproteinase (TIMP)-1, vascular cell adhesion molecule (VCAM)-1, as well as proinflammatory cytokines IL-1beta, IL-6, and TNF-alpha. []
Q7: What is the current status of DIM in clinical trials for cancer treatment or prevention?
A7: While DIM shows promise as an anticancer agent in preclinical studies, further research is necessary to determine its efficacy and safety in humans. Although not explicitly mentioned in the provided abstracts, ongoing clinical trials are investigating DIM's potential in various cancer types.
Q8: Are there any known challenges in developing DIM as a therapeutic agent?
A8: Despite its potential, several challenges exist in developing DIM as a therapeutic. One challenge relates to its low bioavailability. [] Strategies such as developing novel formulations or delivery systems are needed to overcome this limitation and enhance its therapeutic efficacy. [] Additionally, further research is required to determine optimal dosages, long-term safety profiles, and potential drug-drug interactions in specific populations.
Q9: What are some potential strategies to improve the bioavailability of DIM?
A9: Researchers are exploring various strategies to enhance DIM's bioavailability. One approach involves developing absorption-enhanced formulations using carriers like block copolymers of oxyethylene and oxypropylene. [] These formulations aim to improve DIM's solubility and absorption in the gastrointestinal tract. Another strategy involves encapsulating DIM in nanoparticles or liposomes, which can protect the compound from degradation and improve its delivery to target tissues.
Avertissement et informations sur les produits de recherche in vitro
Veuillez noter que tous les articles et informations sur les produits présentés sur BenchChem sont destinés uniquement à des fins informatives. Les produits disponibles à l'achat sur BenchChem sont spécifiquement conçus pour des études in vitro, qui sont réalisées en dehors des organismes vivants. Les études in vitro, dérivées du terme latin "in verre", impliquent des expériences réalisées dans des environnements de laboratoire contrôlés à l'aide de cellules ou de tissus. Il est important de noter que ces produits ne sont pas classés comme médicaments et n'ont pas reçu l'approbation de la FDA pour la prévention, le traitement ou la guérison de toute condition médicale, affection ou maladie. Nous devons souligner que toute forme d'introduction corporelle de ces produits chez les humains ou les animaux est strictement interdite par la loi. Il est essentiel de respecter ces directives pour assurer la conformité aux normes légales et éthiques en matière de recherche et d'expérimentation.