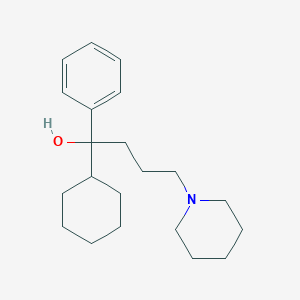
Hexahydrodifenidol
- Cliquez sur DEMANDE RAPIDE pour recevoir un devis de notre équipe d'experts.
- Avec des produits de qualité à un prix COMPÉTITIF, vous pouvez vous concentrer davantage sur votre recherche.
Vue d'ensemble
Description
Hexahydrodifenidol (HHD) is a chiral muscarinic acetylcholine receptor (mAChR) antagonist with selectivity for the M3 receptor subtype. It is structurally characterized by a tertiary amino group and a cyclohexyl-phenyl moiety, which contribute to its receptor-binding properties . HHD is primarily used in pharmacological research to study smooth muscle contraction and airway function, particularly in the context of bronchodilation. The R-enantiomer of HHD (R-HHD) demonstrates significantly higher potency than the S-enantiomer (S-HHD), with racemic mixtures showing reduced efficacy due to antagonistic interactions between enantiomers .
Méthodes De Préparation
Voies de synthèse et conditions réactionnelles : La N-acétyltyramine peut être synthétisée par l’acétylation de la tyramine. La réaction implique généralement l’utilisation d’anhydride acétique ou de chlorure d’acétyle en présence d’une base comme la pyridine ou la triéthylamine. La réaction est effectuée dans des conditions douces, généralement à température ambiante, pour produire la N-acétyltyramine .
Méthodes de production industrielle : La production industrielle de la N-acétyltyramine implique la fermentation de souches spécifiques d’actinobactéries, telles qu’Actinokineospora sp. UTMC 968. Le bouillon de fermentation est ensuite soumis à des processus d’extraction et de purification pour isoler la N-acétyltyramine .
Analyse Des Réactions Chimiques
Types de réactions : La N-acétyltyramine subit diverses réactions chimiques, y compris :
Oxydation : Elle peut être oxydée pour former les quinones correspondantes.
Réduction : Les réactions de réduction peuvent la reconvertir en tyramine.
Substitution : Le groupe hydroxyle de la N-acétyltyramine peut subir des réactions de substitution pour former divers dérivés.
Réactifs et conditions courants :
Oxydation : Les oxydants courants comprennent le permanganate de potassium et le peroxyde d’hydrogène.
Réduction : Des agents réducteurs tels que le borohydrure de sodium ou l’hydrure de lithium et d’aluminium sont utilisés.
Substitution : Des réactifs comme les halogénures d’alkyle ou les chlorures d’acyle sont utilisés en conditions basiques.
Principaux produits :
Oxydation : Quinones.
Réduction : Tyramine.
Substitution : Divers dérivés N-substitués.
4. Applications de la recherche scientifique
La N-acétyltyramine a une large gamme d’applications dans la recherche scientifique :
Chimie : Elle est utilisée comme précurseur dans la synthèse de divers composés bioactifs.
Biologie : Elle sert d’outil pour étudier le rôle des tyramines dans les systèmes biologiques.
Médecine : En raison de ses activités antitumorales et fongitoxices, elle est explorée pour des applications thérapeutiques potentielles.
Industrie : Elle est utilisée dans la production de produits pharmaceutiques et comme réactif biochimique
Applications De Recherche Scientifique
Pharmacological Properties
Hexahydrodifenidol is structurally related to other muscarinic antagonists and exhibits varying affinities for different muscarinic receptor subtypes (M1, M2, M3, and M4). Research indicates that:
- Binding Affinity : The (R)-enantiomer of this compound shows significantly higher affinity for M1 receptors (pKi 7.9 to 8.3) compared to M2 receptors (pKi 7.0) and is less selective than its (S)-counterpart, which has lower overall affinity (pKi 5.8 to 6.1) .
- Stereoselectivity : The stereochemistry of HHD plays a crucial role in its receptor selectivity. The (R)-isomer has been shown to bind more effectively to M1 and M4 receptors than to M2 and M3 receptors, indicating a potential for targeted therapeutic applications .
Neuropharmacology
This compound's ability to selectively bind to muscarinic receptors makes it a valuable tool in neuropharmacological studies aimed at understanding cholinergic signaling pathways. It has been utilized in experiments investigating:
- Receptor Mechanisms : Studies have demonstrated how modifications to the HHD structure affect binding affinities and receptor interactions, providing insights into the molecular mechanisms underlying receptor activation and inhibition .
- Thermodynamic Studies : Research has explored the thermodynamics of HHD binding, revealing that binding affinities can be influenced by temperature and structural variations, which can inform drug design strategies .
Potential Therapeutic Uses
Given its pharmacological profile, this compound may have implications in treating conditions associated with dysregulated cholinergic activity:
- Cognitive Disorders : Due to its selectivity for M1 receptors, HHD may be investigated for potential benefits in cognitive enhancement or treatment of disorders like Alzheimer's disease, where cholinergic dysfunction is prevalent .
- Cardiovascular Applications : Its interaction with cardiac M2 receptors suggests possible applications in managing cardiovascular diseases by modulating heart rate and contractility through muscarinic pathways .
Binding Affinity Studies
A significant study evaluated the binding affinities of various HHD analogs at different muscarinic receptor subtypes. Results indicated that replacing specific moieties within the HHD structure could enhance selectivity towards certain receptors while diminishing affinity towards others. For example:
Compound | M1 Binding Affinity (pKi) | M2 Binding Affinity (pKi) | Notes |
---|---|---|---|
(R)-HHD | 7.9 | 7.0 | Higher selectivity for M1 |
(S)-HHD | 5.8 | 5.9 | Non-selective |
Modified Analog | Varies | Varies | Structural modifications studied |
This table illustrates how structural changes can impact receptor interactions, guiding future drug development efforts.
Therapeutic Exploration
Another study examined the effects of HHD on cognitive function in animal models. The findings suggested that administration of HHD improved memory retention in tasks reliant on cholinergic signaling, supporting further investigation into its use as a cognitive enhancer.
Mécanisme D'action
La N-acétyltyramine exerce ses effets par le biais de plusieurs mécanismes :
Antagonisme du récepteur de l’interleukine-1 : Elle inhibe la liaison de l’interleukine-1 à son récepteur, modulant ainsi les réponses inflammatoires.
Inhibition du facteur XIIIa : Elle inhibe l’activité du facteur XIIIa, qui joue un rôle dans la coagulation sanguine.
Activité antitumorale : Elle induit l’apoptose dans les cellules cancéreuses par le biais de diverses voies moléculaires.
Composés similaires :
N-acétyltryptamine : Un autre alcaloïde avec des bioactivités similaires.
Tyramine : Le composé parent de la N-acétyltyramine.
N-acétylphénéthylamine : Un composé structurellement apparenté avec des bioactivités différentes.
Unicité : La N-acétyltyramine est unique en raison de son double rôle d’antagoniste du récepteur de l’interleukine-1 et d’inhibiteur du facteur XIIIa. Cette double activité n’est pas couramment observée dans d’autres composés similaires, ce qui fait de la N-acétyltyramine un composé précieux pour la recherche thérapeutique .
Comparaison Avec Des Composés Similaires
Enantiomeric Potency and Receptor Selectivity
HHD is part of a class of chiral M3 antagonists that includes trihexyphenidyl, p-fluorohexahydrodifenidol, and p-fluorohexbutinol. Key findings:
- Potency Ratios: R-HHD exhibits an IC50 of 3.4 nM for M3 receptors in rabbit trachea, compared to S-HHD (IC50 = 980 nM), yielding a potency ratio (S/R) of 288 . Similarly, p-fluorohexbutinol shows a potency ratio of 6 (S/R), while trihexyphenidyl has a ratio of 288 . Pfeiffer’s Rule: Higher eutomer (R-enantiomer) potency correlates with increased enantiomeric discrimination, as seen in these compounds .
Compound | IC50 (R-enantiomer, nM) | IC50 (S-enantiomer, nM) | Potency Ratio (S/R) |
---|---|---|---|
This compound | 3.4 | 980 | 288 |
p-Fluorohexbutinol | 12 | 72 | 6 |
Trihexyphenidyl | 0.8 | 230 | 288 |
- Racemic Mixture Effects : The racemic HHD mixture has lower potency than pure R-HHD, as S-HHD acts as a competitive distomer, reducing R-HHD efficacy by 30–50% .
Structural Analogs and Bioisosteric Replacements
Hexahydrosiladifenidol (HHSiD)
- Structure : Silicon replaces carbon in the cyclohexyl group (C/Si bioisosterism).
- Receptor Affinity : Retains M3 selectivity but shows altered pharmacokinetics. p-Fluoro-HHSiD (p-F-HHSiD) has a pKi of 7.8 for M3 receptors, comparable to HHD .
- Therapeutic Potential: Improved metabolic stability due to silicon’s electronegativity and steric effects .
Cyclic Amidines
- Replacing HHD’s tertiary amino group with a cyclic amidine moiety (e.g., M-4 and M-5 analogs) enhances M3 affinity (KD = 0.5–1.2 nM) and smooth muscle selectivity, suggesting utility as antispasmodics .
Comparison with Broader mAChR Antagonists
Compound | M3 pKi | M2 pKi | Selectivity (M3/M2) | Key Application |
---|---|---|---|---|
This compound | 7.65 | 6.75 | 10.0 | Bronchodilation research |
4-DAMP | 8.41 | 7.95 | 2.9 | Broad mAChR inhibition |
Pirenzepine | 6.44 | 6.20 | 1.7 | M1-selective GI disorders |
Methoctramine | 8.22 | 8.09 | 1.3 | Cardiac M2 modulation |
- Key Insights :
Therapeutic Implications
Activité Biologique
Hexahydrodifenidol (HHD) is a compound of significant interest in pharmacology, particularly for its interactions with muscarinic acetylcholine receptors. This article explores the biological activity of HHD, focusing on its receptor binding profiles, pharmacological properties, and structural analogues. A detailed analysis of case studies and research findings will be presented to provide a comprehensive understanding of HHD's biological relevance.
Receptor Binding Affinity
HHD is primarily known for its antagonistic effects on muscarinic receptors, which are vital for various physiological processes. Studies have shown that HHD exhibits different affinities for the muscarinic receptor subtypes M1, M2, and M3:
- M1 Receptors : Highest affinity observed.
- M3 Receptors : Intermediate affinity.
- M2 Receptors : Lowest affinity.
This selectivity profile indicates that HHD can be a valuable tool in studying muscarinic receptor functions and their implications in physiological responses .
Stereoselectivity
Research highlights the importance of stereochemistry in the binding efficacy of HHD. The (R) and (S) enantiomers of HHD display distinct binding affinities across the muscarinic receptor subtypes:
- (R)-HHD : Exhibits higher affinity at M1 receptors compared to M2 and M3.
- (S)-HHD : Shows varied affinities, with lower potency overall.
This stereoselectivity emphasizes the necessity for careful consideration of compound chirality in drug development targeting muscarinic receptors .
Structural Analogs and Their Activity
A series of structural analogs of HHD have been synthesized to evaluate their pharmacological properties. Notable findings include:
Compound | M1 Affinity (pA2) | M2 Affinity (pA2) | M3 Affinity (pA2) |
---|---|---|---|
Hexahydro-difenidol (HHD) | 6.68 | 6.01 | 7.84 |
p-Fluoro-Hexahydro-difenidol | 7.50 | 6.30 | 8.00 |
Hexahydro-sila-difenidol | 7.00 | 5.90 | 7.70 |
These data illustrate that modifications to the phenyl ring or amino group can significantly alter receptor selectivity and binding affinity, providing insights into the design of more effective muscarinic antagonists .
Case Study 1: Antagonistic Effects on Smooth Muscle
In a study examining the effects of HHD on smooth muscle contractions, it was found that HHD effectively inhibited contractions induced by acetylcholine in guinea-pig ileum preparations. The inhibition was dose-dependent and confirmed the compound's role as a competitive antagonist at M3 receptors, supporting its potential therapeutic applications in conditions characterized by excessive cholinergic activity .
Case Study 2: Stereoselective Pharmacology
Another investigation focused on the stereoselective pharmacology of HHD enantiomers demonstrated that (R)-HHD was more effective than (S)-HHD in inhibiting acetylcholine-induced responses in rat atria. This study underscored the importance of stereochemistry in modulating the pharmacological profile of muscarinic antagonists and highlighted the potential for developing targeted therapies based on specific enantiomeric forms .
Q & A
Basic Research Questions
Q. How can researchers determine the receptor selectivity profile of HHD in muscarinic acetylcholine receptor (mAChR) subtypes?
- Methodological Answer : Receptor binding assays using radioligands (e.g., [³H]-N-methylscopolamine) on transfected cell lines expressing M1–M5 mAChR subtypes are critical. Competitive inhibition curves and IC₅₀ calculations should be compared across subtypes. For functional activity, measure HHD’s effect on second messengers (e.g., IP₃ accumulation for M1/M3 or cAMP inhibition for M2/M4). Cross-validate results with selective antagonists like pirenzepine (M1) or AF-DX 116 (M2) to confirm specificity .
Q. What experimental design considerations are essential for assessing HHD’s anticholinergic effects in vivo?
- Methodological Answer : Use animal models (e.g., guinea pig ileum for M3-mediated contractions or murine salivary secretion assays). Include dose-response studies, control groups (vehicle and positive controls like atropine), and blinded data collection. Measure both peripheral and central effects via intraperitoneal vs. intracerebroventricular administration. Ensure compliance with ethical guidelines for humane endpoints .
Q. How should researchers address discrepancies between in vitro binding affinity and in vivo efficacy data for HHD?
- Methodological Answer : Evaluate pharmacokinetic factors (e.g., blood-brain barrier penetration using logP calculations or in situ perfusion models). Compare tissue-specific metabolism via liver microsomal assays. Use techniques like microdialysis to measure free HHD concentrations in target tissues. Confounding variables (e.g., endogenous acetylcholine levels) should be controlled .
Advanced Research Questions
Q. What strategies resolve contradictions in HHD’s reported potency across studies?
- Methodological Answer : Conduct meta-analyses of published IC₅₀ values, adjusting for assay conditions (e.g., buffer pH, temperature). Validate findings using orthogonal methods (e.g., calcium imaging for functional activity vs. radioligand binding). Explore species-specific receptor polymorphisms via sequence alignment and site-directed mutagenesis .
Q. How can bioisosteric replacements (e.g., silicon-for-carbon) optimize HHD’s pharmacological profile?
- Methodological Answer : Synthesize analogs like hexahydrosiladifenidol (HHSiD) and compare steric/electronic properties using computational tools (e.g., DFT calculations). Assess metabolic stability via cytochrome P450 inhibition assays. Use crystallography or cryo-EM to map analog-receptor interactions and identify structural determinants of selectivity .
Q. What analytical methods are required to characterize HHD’s enantiomeric purity and its impact on receptor binding?
- Methodological Answer : Employ chiral chromatography (e.g., Chiralpak AD-H column) with polarimetric detection. Compare enantiomers’ binding kinetics (kₒₙ/kₒff) using surface plasmon resonance (SPR). For in vivo relevance, conduct stereoselective pharmacokinetic studies in rodent models .
Q. How should researchers mitigate HHD’s aquatic toxicity risks in laboratory waste disposal?
- Methodological Answer : Follow OSHA and EPA guidelines for hazardous waste. Use adsorption techniques (e.g., activated charcoal) to treat aqueous waste. Quantify residual HHD via LC-MS/MS before disposal. Document protocols in institutional chemical hygiene plans .
Q. Data Analysis & Reporting
Q. What statistical models are appropriate for analyzing HHD’s dose-dependent effects?
- Methodological Answer : Use nonlinear regression (e.g., log[inhibitor] vs. response in GraphPad Prism) to calculate EC₅₀/IC₅₀. For multi-variable datasets (e.g., receptor subtype comparisons), apply ANOVA with post-hoc Tukey tests. Report confidence intervals and effect sizes to contextualize significance .
Q. How can cross-species extrapolation of HHD’s effects be validated?
- Methodological Answer : Perform comparative genomics on mAChR sequences from human, rodent, and non-rodent models. Use ex vivo organ baths (e.g., human bronchial smooth muscle) to confirm translational relevance. Incorporate species-specific allometric scaling in pharmacokinetic modeling .
Q. What methodologies identify HHD’s off-target interactions in complex biological systems?
- Methodological Answer : Use high-throughput screening panels (e.g., CEREP’s Psychoactive Drug Screen) or proteome-wide affinity chromatography. Combine transcriptomics (RNA-seq) and cheminformatics (SwissTargetPrediction) to map putative targets. Validate hits with CRISPR knockout models .
Q. Tables for Quick Reference
Propriétés
Numéro CAS |
113010-69-8 |
---|---|
Formule moléculaire |
C21H33NO |
Poids moléculaire |
315.5 g/mol |
Nom IUPAC |
1-cyclohexyl-1-phenyl-4-piperidin-1-ylbutan-1-ol |
InChI |
InChI=1S/C21H33NO/c23-21(19-11-4-1-5-12-19,20-13-6-2-7-14-20)15-10-18-22-16-8-3-9-17-22/h1,4-5,11-12,20,23H,2-3,6-10,13-18H2 |
Clé InChI |
ILHSFCNKNNNPRN-UHFFFAOYSA-N |
SMILES |
C1CCC(CC1)C(CCCN2CCCCC2)(C3=CC=CC=C3)O |
SMILES canonique |
C1CCC(CC1)C(CCCN2CCCCC2)(C3=CC=CC=C3)O |
Synonymes |
hexahydro-diphenidol hexahydrodifenidol hexahydrodiphenidol |
Origine du produit |
United States |
Avertissement et informations sur les produits de recherche in vitro
Veuillez noter que tous les articles et informations sur les produits présentés sur BenchChem sont destinés uniquement à des fins informatives. Les produits disponibles à l'achat sur BenchChem sont spécifiquement conçus pour des études in vitro, qui sont réalisées en dehors des organismes vivants. Les études in vitro, dérivées du terme latin "in verre", impliquent des expériences réalisées dans des environnements de laboratoire contrôlés à l'aide de cellules ou de tissus. Il est important de noter que ces produits ne sont pas classés comme médicaments et n'ont pas reçu l'approbation de la FDA pour la prévention, le traitement ou la guérison de toute condition médicale, affection ou maladie. Nous devons souligner que toute forme d'introduction corporelle de ces produits chez les humains ou les animaux est strictement interdite par la loi. Il est essentiel de respecter ces directives pour assurer la conformité aux normes légales et éthiques en matière de recherche et d'expérimentation.