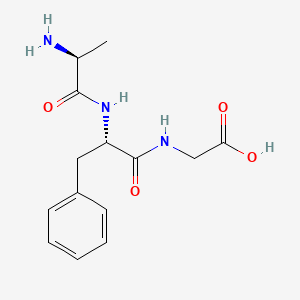
H-Ala-Phe-Gly-OH
Vue d'ensemble
Description
Applications De Recherche Scientifique
H-Ala-Phe-Gly-OH has a wide range of scientific research applications, including:
Chemistry: It is used as a model compound to study peptide synthesis and reactions.
Biology: It is used to study protein-protein interactions and enzyme-substrate interactions.
Safety and Hazards
When handling “H-Ala-Phe-Gly-OH”, it is recommended to avoid dust formation, avoid breathing mist, gas or vapours, avoid contacting with skin and eye, use personal protective equipment, wear chemical impermeable gloves, ensure adequate ventilation, remove all sources of ignition, evacuate personnel to safe areas, and keep people away from and upwind of spill/leak .
Orientations Futures
Peptides like “H-Ala-Phe-Gly-OH” have gained widespread popularity in the field of nanomedicine . They have found a range of applications, from drug delivery and biomaterials to new therapeutic paradigms . In the future, there is a need to make peptide synthesis greener, which can be achieved through solvent substitution, recycling and reduction, as well as exploring alternative synthetic methods .
Méthodes De Préparation
Synthetic Routes and Reaction Conditions
The synthesis of H-Ala-Phe-Gly-OH typically involves solid-phase peptide synthesis (SPPS), which is a widely used method for the chemical synthesis of peptides. SPPS allows for the routine synthesis of virtually any type of peptide sequence, including complex or cyclic peptide products . The process involves the sequential addition of protected amino acids to a growing peptide chain anchored to a solid support. The protecting groups are removed under specific conditions to allow for the formation of peptide bonds.
Industrial Production Methods
In industrial settings, the production of peptides like this compound often involves large-scale SPPS. This method is scalable and can be automated, making it suitable for the production of peptide-based drugs . Efforts are also being made to make peptide synthesis greener by reducing solvent use and exploring alternative synthetic methods .
Analyse Des Réactions Chimiques
Types of Reactions
H-Ala-Phe-Gly-OH can undergo various chemical reactions, including:
Oxidation: This reaction involves the addition of oxygen or the removal of hydrogen. Common oxidizing agents include hydrogen peroxide and potassium permanganate.
Reduction: This reaction involves the addition of hydrogen or the removal of oxygen. Common reducing agents include sodium borohydride and lithium aluminum hydride.
Substitution: This reaction involves the replacement of one functional group with another. Common reagents include alkyl halides and nucleophiles.
Common Reagents and Conditions
The reactions of this compound typically require specific reagents and conditions. For example, oxidation reactions may require acidic or basic conditions, while reduction reactions may require anhydrous conditions to prevent the hydrolysis of the reducing agent .
Major Products Formed
The major products formed from these reactions depend on the specific reagents and conditions used. For example, oxidation of this compound may result in the formation of corresponding oxo derivatives, while reduction may yield the corresponding alcohols .
Mécanisme D'action
The mechanism of action of H-Ala-Phe-Gly-OH involves its interaction with specific molecular targets and pathways. For example, it may interact with enzymes involved in peptide metabolism, leading to the formation of bioactive peptides. The specific pathways involved depend on the biological context and the specific application of the compound .
Comparaison Avec Des Composés Similaires
H-Ala-Phe-Gly-OH can be compared with other similar compounds, such as:
Gly-Phe: A dipeptide composed of glycine and phenylalanine.
Phe-Phe: A dipeptide composed of two phenylalanine residues.
These compounds share similar structural features but differ in their specific amino acid sequences and properties. This compound is unique due to its specific tripeptide sequence, which may confer distinct biological and chemical properties .
Propriétés
IUPAC Name |
2-[[(2S)-2-[[(2S)-2-aminopropanoyl]amino]-3-phenylpropanoyl]amino]acetic acid | |
---|---|---|
Details | Computed by Lexichem TK 2.7.0 (PubChem release 2021.05.07) | |
Source | PubChem | |
URL | https://pubchem.ncbi.nlm.nih.gov | |
Description | Data deposited in or computed by PubChem | |
InChI |
InChI=1S/C14H19N3O4/c1-9(15)13(20)17-11(14(21)16-8-12(18)19)7-10-5-3-2-4-6-10/h2-6,9,11H,7-8,15H2,1H3,(H,16,21)(H,17,20)(H,18,19)/t9-,11-/m0/s1 | |
Details | Computed by InChI 1.0.6 (PubChem release 2021.05.07) | |
Source | PubChem | |
URL | https://pubchem.ncbi.nlm.nih.gov | |
Description | Data deposited in or computed by PubChem | |
InChI Key |
DHBKYZYFEXXUAK-ONGXEEELSA-N | |
Details | Computed by InChI 1.0.6 (PubChem release 2021.05.07) | |
Source | PubChem | |
URL | https://pubchem.ncbi.nlm.nih.gov | |
Description | Data deposited in or computed by PubChem | |
Canonical SMILES |
CC(C(=O)NC(CC1=CC=CC=C1)C(=O)NCC(=O)O)N | |
Details | Computed by OEChem 2.3.0 (PubChem release 2021.05.07) | |
Source | PubChem | |
URL | https://pubchem.ncbi.nlm.nih.gov | |
Description | Data deposited in or computed by PubChem | |
Isomeric SMILES |
C[C@@H](C(=O)N[C@@H](CC1=CC=CC=C1)C(=O)NCC(=O)O)N | |
Details | Computed by OEChem 2.3.0 (PubChem release 2021.05.07) | |
Source | PubChem | |
URL | https://pubchem.ncbi.nlm.nih.gov | |
Description | Data deposited in or computed by PubChem | |
Molecular Formula |
C14H19N3O4 | |
Details | Computed by PubChem 2.1 (PubChem release 2021.05.07) | |
Source | PubChem | |
URL | https://pubchem.ncbi.nlm.nih.gov | |
Description | Data deposited in or computed by PubChem | |
Molecular Weight |
293.32 g/mol | |
Details | Computed by PubChem 2.1 (PubChem release 2021.05.07) | |
Source | PubChem | |
URL | https://pubchem.ncbi.nlm.nih.gov | |
Description | Data deposited in or computed by PubChem | |
Retrosynthesis Analysis
AI-Powered Synthesis Planning: Our tool employs the Template_relevance Pistachio, Template_relevance Bkms_metabolic, Template_relevance Pistachio_ringbreaker, Template_relevance Reaxys, Template_relevance Reaxys_biocatalysis model, leveraging a vast database of chemical reactions to predict feasible synthetic routes.
One-Step Synthesis Focus: Specifically designed for one-step synthesis, it provides concise and direct routes for your target compounds, streamlining the synthesis process.
Accurate Predictions: Utilizing the extensive PISTACHIO, BKMS_METABOLIC, PISTACHIO_RINGBREAKER, REAXYS, REAXYS_BIOCATALYSIS database, our tool offers high-accuracy predictions, reflecting the latest in chemical research and data.
Strategy Settings
Precursor scoring | Relevance Heuristic |
---|---|
Min. plausibility | 0.01 |
Model | Template_relevance |
Template Set | Pistachio/Bkms_metabolic/Pistachio_ringbreaker/Reaxys/Reaxys_biocatalysis |
Top-N result to add to graph | 6 |
Feasible Synthetic Routes
Q1: What is the significance of the H-Ala-Phe-Gly-OH sequence in opioid peptide research?
A1: The this compound sequence, often found within larger peptides, plays a crucial role in opioid peptide research. While not inherently bioactive on its own, it serves as a framework for modifications that can dramatically influence the overall peptide's activity, potency, and selectivity towards different opioid receptors (mu, delta, kappa). [, , , , , , ]
Q2: How does the substitution of Glycine in the this compound sequence with other amino acids or moieties affect the activity of dermorphin analogues?
A2: Replacing Glycine in the this compound sequence, specifically within the context of dermorphin analogues, significantly impacts their opioid activity. Studies have explored substitutions with various amino acids like sarcosine and modifications like the ketomethylene linkage. These alterations often result in reduced opioid activity compared to the parent peptide, highlighting the importance of Glycine in maintaining the structural integrity required for optimal receptor interactions. [, ]
Q3: What is the role of the this compound sequence in the selectivity of opioid peptides towards mu or delta opioid receptors?
A3: The this compound sequence itself does not dictate selectivity towards mu or delta opioid receptors. This selectivity arises from the influence of flanking amino acid sequences within the larger peptide structure. For instance, in dermorphin, a mu-selective opioid, the presence of the C-terminal Tyr-Pro-Ser-NH2 sequence contributes to its mu receptor preference. [, , ]
Q4: How does the incorporation of D-amino acids, such as D-Alanine, in the this compound sequence impact the activity and stability of opioid peptides?
A4: Incorporating D-amino acids like D-Alanine into the this compound sequence, as observed in dermorphin and related peptides, is crucial for both their potent activity and enhanced stability. The presence of D-Alanine in the second position of dermorphin is essential for its high potency and selectivity for mu-opioid receptors. Moreover, the use of D-amino acids can increase resistance to enzymatic degradation, leading to a longer duration of action. [, , ]
Q5: Can you elaborate on the importance of the relative positioning of the tyrosine and phenylalanine residues within the this compound sequence and its impact on opioid activity?
A5: The precise spatial arrangement of the tyrosine and phenylalanine residues within the this compound sequence is crucial for opioid activity. These aromatic rings, particularly tyrosine at the N-terminal, are recognized as key pharmacophoric elements essential for interacting with opioid receptors. Modifications altering their relative positions or orientations can significantly impact a peptide's binding affinity and subsequent downstream effects. [, , ]
Q6: How does the introduction of conformational constraints, such as those achieved through cyclization or the use of specific amino acid substitutions, affect the biological activity of peptides containing the this compound sequence?
A6: Introducing conformational constraints significantly impacts the biological activity of peptides containing the this compound sequence. Techniques like cyclization or incorporating specific amino acid substitutions can restrict the peptide's flexibility, forcing it into a specific conformation. This can lead to enhanced selectivity for certain opioid receptors, increased potency, and improved resistance to enzymatic degradation, ultimately influencing the peptide's overall pharmacological profile. [, ]
Q7: How do chemical modifications, such as the introduction of fluorine atoms or sulfate groups, influence the binding affinity and selectivity of peptides containing the this compound sequence for opioid receptors?
A7: Chemical modifications, such as adding fluorine atoms or sulfate groups, can significantly alter the pharmacological properties of peptides containing the this compound sequence. For example, direct electrophilic fluorination of tyrosine residues in dermorphin analogues leads to a decrease in both mu- and delta-opioid receptor affinities, albeit without significantly impacting their selectivity for mu-opioid receptors. [] Similarly, incorporating tyrosine sulfate into dermorphin and deltorphin peptides differentially affects their activity. While the sulfated dermorphin analogue shows minimal interaction with opioid receptors, the sulfated deltorphin analogue retains significant activity, indicating a complex interplay between modification and peptide structure that influences receptor interactions. []
Q8: How have computational chemistry techniques, like molecular dynamics simulations, contributed to our understanding of the conformational preferences and interactions of peptides containing the this compound sequence with opioid receptors?
A8: Computational chemistry, particularly molecular dynamics simulations, has been instrumental in deciphering the structural dynamics and receptor interactions of peptides containing the this compound sequence. These simulations, often guided by experimental data like NMR restraints, provide valuable insights into the conformational preferences of these peptides in various environments, including membrane-mimetic systems. They help visualize potential binding modes with opioid receptors, identify key pharmacophoric elements, and predict the impact of structural modifications on binding affinities, thus aiding in the design and optimization of novel opioid ligands. [, , , ]
Avertissement et informations sur les produits de recherche in vitro
Veuillez noter que tous les articles et informations sur les produits présentés sur BenchChem sont destinés uniquement à des fins informatives. Les produits disponibles à l'achat sur BenchChem sont spécifiquement conçus pour des études in vitro, qui sont réalisées en dehors des organismes vivants. Les études in vitro, dérivées du terme latin "in verre", impliquent des expériences réalisées dans des environnements de laboratoire contrôlés à l'aide de cellules ou de tissus. Il est important de noter que ces produits ne sont pas classés comme médicaments et n'ont pas reçu l'approbation de la FDA pour la prévention, le traitement ou la guérison de toute condition médicale, affection ou maladie. Nous devons souligner que toute forme d'introduction corporelle de ces produits chez les humains ou les animaux est strictement interdite par la loi. Il est essentiel de respecter ces directives pour assurer la conformité aux normes légales et éthiques en matière de recherche et d'expérimentation.