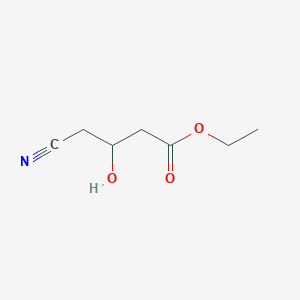
Ethyl 4-cyano-3-hydroxybutanoate
Vue d'ensemble
Description
Ethyl (R)-4-cyano-3-hydroxybutanoate (CAS 141942-85-0, EINECS 430-220-6) is a chiral ester with the molecular formula C₇H₁₁NO₃ and a molecular weight of 157.17 g/mol . It features a cyano (-CN) group at the 4th position and a hydroxyl (-OH) group at the 3rd position on the butanoate backbone. This compound is a critical intermediate in synthesizing atorvastatin, a blockbuster cholesterol-lowering drug, due to its enantioselective biocatalytic production via engineered halohydrin dehalogenases (HHDHs) like HheC2360 . Its (R)-configuration is essential for pharmaceutical efficacy, necessitating precise enzymatic methods to avoid racemic mixtures .
Applications De Recherche Scientifique
Ethyl 4-cyano-3-hydroxybutanoate is a versatile compound with applications in pharmaceutical development, biochemical research, agrochemical formulations, cosmetic applications, and the food industry . It exists in two enantiomeric forms, (R)-ethyl 4-cyano-3-hydroxybutanoate and (S)-ethyl 4-cyano-3-hydroxybutanoate .
Pharmaceutical Development
Ethyl (R)-(-)-4-cyano-3-hydroxybutyrate serves as an intermediate in synthesizing pharmaceuticals, especially in developing drugs targeting metabolic disorders . It is also a crucial intermediate in the synthesis of Hydroxyglutaryl coenzyme A (HMG-CoA) reductase inhibitors, a class of lipid-lowering drugs with a significant market share . These inhibitors reduce cholesterol synthesis in the liver, increase low-density lipoprotein (LDL) cholesterol receptors, and lower plasma cholesterol levels . Synthetic atorvastatin, derived from ethyl (R)-(-)-4-cyano-3-hydroxybutyrate, is used to treat hypercholesterolemia, mixed hyperlipidemia, coronary heart disease, and stroke .
Biochemical Research
This compound is utilized in studies of metabolic pathways, helping researchers understand the roles of specific enzymes and substrates in biological systems .
Agrochemical Formulations
Ethyl (R)-(-)-4-cyano-3-hydroxybutyrate can be integrated into agrochemical products to enhance the effectiveness of herbicides and pesticides by improving their absorption and activity in plants .
Cosmetic Applications
The potential of Ethyl (R)-(-)-4-cyano-3-hydroxybutyrate is explored in skincare formulations for its moisturizing and anti-aging properties .
Food Industry
This compound may be used as a food additive or flavoring agent, providing unique flavor profiles while adhering to safety and regulatory standards .
Mécanisme D'action
Ethyl 4-cyano-3-hydroxybutanoate can be compared with other similar compounds such as:
- Ethyl 4-chloro-3-hydroxybutanoate
- Ethyl 4-bromo-3-hydroxybutanoate
- Ethyl 4-azido-3-hydroxybutanoate
Uniqueness:
Comparaison Avec Des Composés Similaires
Comparison with Structurally Similar Compounds
Ethyl 3-Hydroxybutanoate
Molecular Formula : C₆H₁₂O₃
Molecular Weight : 132.16 g/mol
Key Differences :
- Substituents: Lacks the cyano group at position 4.
- Synthesis: Produced via yeast-mediated reduction of ethyl acetoacetate, a simpler process compared to the HHDH-catalyzed cyanolysis required for ethyl 4-cyano-3-hydroxybutanoate .
- Applications: Primarily used in flavorings and fragrances due to its fruity odor, unlike the pharmaceutical focus of its cyano-substituted analog .
Property | This compound | Ethyl 3-Hydroxybutanoate |
---|---|---|
Boiling Point (°C) | 325.6 (predicted) | Not reported |
LogP | -0.24 | 0.34 (predicted) |
Key Functional Groups | -CN, -OH | -OH |
Research Insight: The cyano group in this compound significantly lowers its LogP (-0.24 vs. 0.34), enhancing hydrophilicity and altering metabolic stability, which is critical for drug intermediates .
Ethyl 4-Chloro-3-Hydroxybutanoate
Molecular Formula : C₆H₁₁ClO₃
Molecular Weight : 166.60 g/mol
Key Differences :
- Substituents: Chloro (-Cl) replaces the cyano (-CN) group.
- Reactivity: The chloro group acts as a leaving group, enabling nucleophilic substitution reactions, whereas the cyano group facilitates nitrile-specific transformations (e.g., hydrolysis to carboxylic acids) .
- Safety: Chlorinated analogs often exhibit higher toxicity, requiring stringent handling protocols compared to the cyano variant .
(S)-4-Cyano-3-Hydroxybutanoate Ethyl Ester
Molecular Formula: C₇H₁₁NO₃ Molecular Weight: 157.17 g/mol Key Differences:
- Stereochemistry : The (S)-enantiomer (CAS 312745-91-8) is pharmacologically inactive in atorvastatin synthesis, underscoring the necessity of enantioselective biocatalysis for the (R)-form .
- Synthesis Challenges : Achieving high enantiomeric excess (ee) for the (S)-form requires distinct enzymatic or chemical resolution methods, which are less optimized than those for the (R)-isomer .
Biocatalytic Efficiency
Directed evolution of HHDHs (e.g., variant V84G/W86F) improved the space-time productivity of ethyl (R)-4-cyano-3-hydroxybutanoate by 2-fold compared to wild-type enzymes, a feat unmatched in synthesizing non-cyano analogs like ethyl 3-hydroxybutanoate .
Regulatory and Industrial Relevance
- Pricing and Availability: this compound is priced at a premium due to its pharmaceutical demand, while ethyl 3-hydroxybutanoate is cheaper and widely available for industrial use .
- Regulatory Status: The cyano compound faces stricter regulatory scrutiny (e.g., FDA guidelines for chiral purity), whereas non-pharmaceutical analogs like ethyl 4-chloro-3-hydroxybutanoate are regulated primarily for safety .
Activité Biologique
Ethyl 4-cyano-3-hydroxybutanoate (also known as ethyl (R)-4-cyano-3-hydroxybutanoate) is a significant compound in pharmaceutical chemistry, primarily recognized as an important chiral synthon for the synthesis of various bioactive molecules, including statins like atorvastatin (Lipitor). This article explores its biological activity, synthesis methods, and relevant research findings.
This compound is characterized by its chiral center and functional groups that contribute to its reactivity. The compound can be synthesized through various methods, including enzymatic and chemical approaches.
Synthesis Methods
-
Enzymatic Synthesis:
- The use of halohydrin dehalogenase (HHDH) from Parvibaculum lavamentivorans has been reported to achieve high conversion rates. In one study, a concentration of 200 g/L of substrate resulted in a 95% conversion rate and an 85% yield within 14 hours .
- Another method involved immobilized halohydrin dehalogenase, which enhanced enzyme stability and recovery rates, leading to yields exceeding 91% .
- Chemical Synthesis:
Biological Activity
This compound exhibits several biological activities that make it valuable in medicinal chemistry:
- Cholesterol-Lowering Effects: It serves as a precursor for atorvastatin, a drug that inhibits hydroxymethylglutaryl-CoA reductase, effectively lowering cholesterol levels in patients .
- Synthesis of Other Bioactive Compounds: This compound is also utilized in the production of L-carnitine and other amino acids, highlighting its versatility in biochemical applications .
Case Studies and Experimental Data
- Enzymatic Activity:
- Industrial Applications:
Data Table: Synthesis Yields and Conditions
Method | Substrate Concentration | Conversion Rate | Yield (%) | Enzyme Used |
---|---|---|---|---|
Enzymatic (HHDH) | 200 g/L | 95% | 85% | HHDH from Parvibaculum lavamentivorans |
Chemical Reaction | Variable | High | >91% | Sodium Cyanide |
Immobilized Enzyme | pH 6.5 - 8.0 | High | >91% | Immobilized Halohydrin Dehalogenase |
Analyse Des Réactions Chimiques
Reaction Conditions
Example Process :
-
At 200 g/L (S)-CHBE, HHDH-PL achieves 95% conversion and 85% yield in 14 h .
-
Immobilized HHDH enables enzyme reuse for 5–10 cycles without significant activity loss .
Epoxide Intermediate Mechanism
The reaction proceeds through an epoxide intermediate:
-
Epoxide Formation : (S)-CHBE is converted to (S)-3,4-epoxybutyrate via HHDH-mediated dehalogenation .
-
Cyanide Ring-Opening : The epoxide reacts with cyanide, yielding HN with >99% ee under controlled pH and temperature .
Key Reaction Pathway
Industrial-Scale Optimization
-
Two-Step Process : Combines ketoreductase-mediated substrate synthesis and HHDH catalysis in one reactor, reducing solvent use by 30% .
-
Purification : Post-reaction, HN is extracted with ethyl acetate, distilled under vacuum, and recrystallized to achieve >99% purity .
Yield Enhancement :
-
Vacuum Decyanation : Removes excess HCN, improving product stability .
-
Immobilized Enzymes : Enable continuous processing with 91% yield at 200 L scale .
Analytical Methods
-
Gas Chromatography (GC) :
Stability and Handling
Q & A
Basic Research Questions
Q. What are the primary synthetic routes for Ethyl 4-cyano-3-hydroxybutanoate (ECHB), and how do they differ in methodology?
ECHB is synthesized via two main approaches:
- Chemical synthesis : Asymmetric reduction of ethyl 4-cyano-3-oxobutanoate using chiral catalysts (e.g., Corey-Bakshi-Shibata reduction) .
- Enzymatic synthesis : Biocatalysis using halohydrin dehalogenases (HHDHs) or carbonyl reductases. For example, Parvibaculum lavamentivorans HHDH-PL converts ethyl (S)-4-chloro-3-hydroxybutanoate to ECHB via enantioselective cyanide substitution . Key differences include enantiomeric excess (ee) (>99% for enzymatic routes vs. ~80-90% for chemical methods) and reliance on NADPH cofactors in enzymatic pathways .
Q. How can researchers verify the enantiomeric purity of ECHB?
Enantiomeric purity is validated using:
- Chiral HPLC : Columns like Chiralpak AD-H or OD-H with hexane/isopropanol mobile phases .
- Polarimetry : Specific rotation comparisons against literature values (e.g., [α]D = +12.5° for (R)-ECHB) .
- NMR with chiral shift reagents : Europium tris[3-(heptafluoropropylhydroxymethylene)-(+)-camphorate] induces diastereomeric splitting .
Advanced Research Questions
Q. What experimental strategies resolve contradictions in catalytic efficiency data for HHDH variants during ECHB synthesis?
Discrepancies in enzyme activity (e.g., kcat variability across HHDH mutants) arise from:
- Substrate inhibition : High substrate concentrations (>200 mM) may reduce activity. Kinetic modeling (e.g., Michaelis-Menten with substrate inhibition terms) clarifies optimal reaction conditions .
- pH dependency : Activity profiles of HHDHs vary significantly between pH 7.0-9.0. Use pH-stat reactors to maintain optimal pH during reactions .
- Structural analysis : Molecular dynamics simulations of HHDH active sites (e.g., W249F mutant) rationalize altered substrate binding .
Q. How do structural modifications of ECHB impact its utility as a chiral synthon in pharmaceutical intermediates?
Comparative studies with analogs reveal:
- Ethyl 4-chloro-3-hydroxybutanoate : Higher reactivity in nucleophilic substitutions but lower enantioselectivity in lipase-catalyzed resolutions .
- Mthis compound : Reduced steric hindrance improves crystallization but lowers thermal stability .
- Ethyl 4-amino-3-hydroxybutanoate : Direct precursor to (R)-GABOB but requires additional protection/deprotection steps . Methodological insights include using QSAR models to predict substitution effects on reaction yields .
Q. What high-throughput screening (HTS) methodologies are effective for engineering ECHB-producing enzymes?
- Azide colorimetric assay : Detects HHDH activity via ε460 = 1.2173 × 10<sup>4</sup> L mol<sup>−1</sup> cm<sup>−1</sup> for azide release from ethyl (S)-4-chloro-3-hydroxybutanoate .
- Microplate-based NADPH depletion assays : Fluorescence quenching (ex/em: 340/460 nm) monitors carbonyl reductase activity .
- Phage display : Screens mutant libraries for improved thermostability (e.g., Tm increases by 8°C in HHDH-PL variants) .
Q. Data Analysis and Validation
Q. How should researchers address discrepancies in reported ee values for ECHB across different studies?
- Standardize analytical conditions : Temperature and solvent composition (e.g., 25°C in hexane/IPA) significantly affect chiral separations .
- Cross-validate with multiple techniques : Combine HPLC, polarimetry, and X-ray crystallography of derivatives (e.g., Mosher esters) .
- Account for kinetic resolution : Early reaction termination in enzymatic syntheses may artificially inflate ee due to incomplete conversion .
Q. What statistical methods are recommended for analyzing enzyme kinetics in ECHB synthesis?
- Nonlinear regression : Fit progress curves to the Henri-Michaelis-Menten equation using tools like GraphPad Prism .
- Error-weighted fitting : Address heteroscedasticity in activity measurements .
- Principal component analysis (PCA) : Correlate mutational hotspots in HHDHs with activity/selectivity changes .
Q. Methodological Resources
Propriétés
IUPAC Name |
ethyl 4-cyano-3-hydroxybutanoate | |
---|---|---|
Details | Computed by LexiChem 2.6.6 (PubChem release 2019.06.18) | |
Source | PubChem | |
URL | https://pubchem.ncbi.nlm.nih.gov | |
Description | Data deposited in or computed by PubChem | |
InChI |
InChI=1S/C7H11NO3/c1-2-11-7(10)5-6(9)3-4-8/h6,9H,2-3,5H2,1H3 | |
Details | Computed by InChI 1.0.5 (PubChem release 2019.06.18) | |
Source | PubChem | |
URL | https://pubchem.ncbi.nlm.nih.gov | |
Description | Data deposited in or computed by PubChem | |
InChI Key |
LOQFROBMBSKWQY-UHFFFAOYSA-N | |
Details | Computed by InChI 1.0.5 (PubChem release 2019.06.18) | |
Source | PubChem | |
URL | https://pubchem.ncbi.nlm.nih.gov | |
Description | Data deposited in or computed by PubChem | |
Canonical SMILES |
CCOC(=O)CC(CC#N)O | |
Details | Computed by OEChem 2.1.5 (PubChem release 2019.06.18) | |
Source | PubChem | |
URL | https://pubchem.ncbi.nlm.nih.gov | |
Description | Data deposited in or computed by PubChem | |
Molecular Formula |
C7H11NO3 | |
Details | Computed by PubChem 2.1 (PubChem release 2019.06.18) | |
Source | PubChem | |
URL | https://pubchem.ncbi.nlm.nih.gov | |
Description | Data deposited in or computed by PubChem | |
DSSTOX Substance ID |
DTXSID20400447 | |
Record name | ethyl 4-cyano-3-hydroxybutanoate | |
Source | EPA DSSTox | |
URL | https://comptox.epa.gov/dashboard/DTXSID20400447 | |
Description | DSSTox provides a high quality public chemistry resource for supporting improved predictive toxicology. | |
Molecular Weight |
157.17 g/mol | |
Details | Computed by PubChem 2.1 (PubChem release 2021.05.07) | |
Source | PubChem | |
URL | https://pubchem.ncbi.nlm.nih.gov | |
Description | Data deposited in or computed by PubChem | |
CAS No. |
227200-13-7 | |
Record name | ethyl 4-cyano-3-hydroxybutanoate | |
Source | EPA DSSTox | |
URL | https://comptox.epa.gov/dashboard/DTXSID20400447 | |
Description | DSSTox provides a high quality public chemistry resource for supporting improved predictive toxicology. | |
Synthesis routes and methods I
Procedure details
Synthesis routes and methods II
Procedure details
Synthesis routes and methods III
Procedure details
Retrosynthesis Analysis
AI-Powered Synthesis Planning: Our tool employs the Template_relevance Pistachio, Template_relevance Bkms_metabolic, Template_relevance Pistachio_ringbreaker, Template_relevance Reaxys, Template_relevance Reaxys_biocatalysis model, leveraging a vast database of chemical reactions to predict feasible synthetic routes.
One-Step Synthesis Focus: Specifically designed for one-step synthesis, it provides concise and direct routes for your target compounds, streamlining the synthesis process.
Accurate Predictions: Utilizing the extensive PISTACHIO, BKMS_METABOLIC, PISTACHIO_RINGBREAKER, REAXYS, REAXYS_BIOCATALYSIS database, our tool offers high-accuracy predictions, reflecting the latest in chemical research and data.
Strategy Settings
Precursor scoring | Relevance Heuristic |
---|---|
Min. plausibility | 0.01 |
Model | Template_relevance |
Template Set | Pistachio/Bkms_metabolic/Pistachio_ringbreaker/Reaxys/Reaxys_biocatalysis |
Top-N result to add to graph | 6 |
Feasible Synthetic Routes
Avertissement et informations sur les produits de recherche in vitro
Veuillez noter que tous les articles et informations sur les produits présentés sur BenchChem sont destinés uniquement à des fins informatives. Les produits disponibles à l'achat sur BenchChem sont spécifiquement conçus pour des études in vitro, qui sont réalisées en dehors des organismes vivants. Les études in vitro, dérivées du terme latin "in verre", impliquent des expériences réalisées dans des environnements de laboratoire contrôlés à l'aide de cellules ou de tissus. Il est important de noter que ces produits ne sont pas classés comme médicaments et n'ont pas reçu l'approbation de la FDA pour la prévention, le traitement ou la guérison de toute condition médicale, affection ou maladie. Nous devons souligner que toute forme d'introduction corporelle de ces produits chez les humains ou les animaux est strictement interdite par la loi. Il est essentiel de respecter ces directives pour assurer la conformité aux normes légales et éthiques en matière de recherche et d'expérimentation.