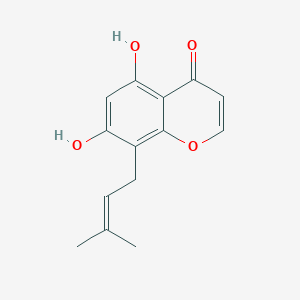
Eriosematin A
Vue d'ensemble
Description
Eriosematin A is a natural product found in Eriosema tuberosum with data available.
Applications De Recherche Scientifique
Antidiarrheal Potential
Eriosematin A, derived from Eriosema chinense Vogel, exhibits significant antidiarrheal properties. Research indicates its effectiveness against diarrhoea induced by enteropathogenic Escherichia coli in rats. The compound's antidiarrheal potential is attributed to its ability to reduce intestinal secretion and restore antioxidant and electrolyte balance, as well as reactivating Na+/K+-ATPase activity, thus preventing epithelial tissue damage (Parmar et al., 2019).
Antifungal Activity
This compound, isolated from Eriosema tuberosum, has shown antifungal activity against Cladosporium cucumerinum and Candida albicans. This antifungal efficacy was determined using TLC bioautographic assays, highlighting its potential in addressing fungal infections (Ma et al., 1996).
Antioxidant Properties
This compound has been explored for its antioxidant activity. Its redox properties were assessed using cyclic voltammetry, and it demonstrated potential in inhibiting lipid peroxidation and protecting serum albumin from oxidation. These findings indicate its role as a natural antioxidant agent (Born et al., 1996).
Mécanisme D'action
Target of Action
Eriosematin A is a compound derived from the Eriosema genus, which includes approximately 150 species widely distributed across tropical and subtropical regions of the world The eriosema genus has been associated with a broad range of activities including aphrodisiac, estrogenic, anti-osteoporosis, hypolipidemic, anti-diabetic, anti-diarrheal, anti-microbial, anti-oxidant, anthelmintic, anti-cancer, and acetylcholinesterase inhibitory activities .
Mode of Action
It is known that the interaction of a drug with its target is related to the drug’s affinity (probability of the drug occupying a receptor at any given instant) and intrinsic efficacy (degree to which a ligand activates receptors and leads to cellular response) . The drug’s affinity and activity are determined by its chemical structure .
Biochemical Pathways
The eriosema genus has been associated with a broad range of pharmacological activities, suggesting that it may interact with multiple biochemical pathways .
Pharmacokinetics
These properties are crucial in determining a drug’s bioavailability . The ADME properties of a drug can be predicted using computational methods .
Result of Action
The eriosema genus has been associated with a broad range of pharmacological activities , suggesting that this compound may have similar effects.
Analyse Biochimique
Biochemical Properties
Eriosematin A plays a significant role in various biochemical reactions. It interacts with several enzymes, proteins, and other biomolecules, influencing their activity and function. For instance, this compound has been shown to inhibit the protease activity of SepA, a protein secreted by Shigella flexneri, by forming dihedral hydrogen bonding with the catalytic triad Serine 211 . This interaction disrupts the protease’s ability to degrade host proteins, thereby mitigating the pathogenic effects of Shigella flexneri . Additionally, this compound exhibits antioxidant properties by scavenging free radicals and reducing oxidative stress in cells .
Cellular Effects
This compound exerts various effects on different cell types and cellular processes. In cancer cells, this compound induces apoptosis, a programmed cell death mechanism, by activating caspase enzymes and promoting the release of cytochrome c from mitochondria . This leads to the activation of downstream apoptotic pathways, resulting in cell death. Furthermore, this compound has been shown to inhibit cell proliferation and induce cell cycle arrest in the G2/M phase . In immune cells, this compound modulates cytokine production and reduces inflammation by inhibiting the nuclear factor-kappa B (NF-κB) signaling pathway .
Molecular Mechanism
The molecular mechanism of this compound involves several key interactions and processes. At the molecular level, this compound binds to specific biomolecules, such as enzymes and receptors, altering their activity and function. For example, this compound inhibits the activity of acetylcholinesterase, an enzyme responsible for the breakdown of acetylcholine, by binding to its active site . This inhibition leads to increased levels of acetylcholine, enhancing cholinergic signaling in the nervous system. Additionally, this compound modulates gene expression by interacting with transcription factors and influencing the transcriptional activity of target genes .
Temporal Effects in Laboratory Settings
In laboratory settings, the effects of this compound have been observed to change over time. The stability and degradation of this compound are critical factors influencing its long-term effects on cellular function. Studies have shown that this compound remains stable under standard laboratory conditions, with minimal degradation over extended periods . Prolonged exposure to light and heat can lead to the breakdown of this compound, reducing its bioactivity . In in vitro and in vivo studies, this compound has demonstrated sustained anti-cancer and anti-inflammatory effects over time, indicating its potential for long-term therapeutic use .
Dosage Effects in Animal Models
The effects of this compound vary with different dosages in animal models. At low doses, this compound exhibits beneficial effects, such as reducing tumor growth and inflammation . At high doses, this compound can induce toxic effects, including hepatotoxicity and nephrotoxicity . Threshold effects have been observed, where the therapeutic benefits of this compound are maximized at specific dosage ranges, beyond which adverse effects become prominent . These findings highlight the importance of optimizing dosage regimens for safe and effective use of this compound in therapeutic applications .
Metabolic Pathways
This compound is involved in several metabolic pathways, interacting with various enzymes and cofactors. One of the key metabolic pathways influenced by this compound is the cytochrome P450 enzyme system . This compound modulates the activity of cytochrome P450 enzymes, affecting the metabolism of other compounds and drugs . Additionally, this compound influences metabolic flux by altering the levels of key metabolites, such as glucose and lipids . These effects on metabolic pathways contribute to the overall bioactivity and therapeutic potential of this compound .
Transport and Distribution
The transport and distribution of this compound within cells and tissues are mediated by specific transporters and binding proteins. This compound is actively transported across cell membranes by ATP-binding cassette (ABC) transporters, facilitating its intracellular accumulation . Once inside the cell, this compound binds to cytoplasmic and nuclear proteins, influencing its localization and activity . The distribution of this compound within tissues is influenced by factors such as blood flow, tissue permeability, and binding affinity to plasma proteins .
Subcellular Localization
This compound exhibits specific subcellular localization, which is crucial for its activity and function. This compound is predominantly localized in the cytoplasm and mitochondria, where it interacts with key enzymes and proteins involved in cellular metabolism and signaling . The targeting of this compound to specific subcellular compartments is facilitated by post-translational modifications, such as phosphorylation and glycosylation, which direct its localization to specific organelles . This subcellular localization is essential for the bioactivity and therapeutic effects of this compound .
Propriétés
IUPAC Name |
5,7-dihydroxy-8-(3-methylbut-2-enyl)chromen-4-one | |
---|---|---|
Details | Computed by LexiChem 2.6.6 (PubChem release 2019.06.18) | |
Source | PubChem | |
URL | https://pubchem.ncbi.nlm.nih.gov | |
Description | Data deposited in or computed by PubChem | |
InChI |
InChI=1S/C14H14O4/c1-8(2)3-4-9-11(16)7-12(17)13-10(15)5-6-18-14(9)13/h3,5-7,16-17H,4H2,1-2H3 | |
Details | Computed by InChI 1.0.5 (PubChem release 2019.06.18) | |
Source | PubChem | |
URL | https://pubchem.ncbi.nlm.nih.gov | |
Description | Data deposited in or computed by PubChem | |
InChI Key |
FQSPUXNQUUIUQW-UHFFFAOYSA-N | |
Details | Computed by InChI 1.0.5 (PubChem release 2019.06.18) | |
Source | PubChem | |
URL | https://pubchem.ncbi.nlm.nih.gov | |
Description | Data deposited in or computed by PubChem | |
Canonical SMILES |
CC(=CCC1=C2C(=C(C=C1O)O)C(=O)C=CO2)C | |
Details | Computed by OEChem 2.1.5 (PubChem release 2019.06.18) | |
Source | PubChem | |
URL | https://pubchem.ncbi.nlm.nih.gov | |
Description | Data deposited in or computed by PubChem | |
Molecular Formula |
C14H14O4 | |
Details | Computed by PubChem 2.1 (PubChem release 2019.06.18) | |
Source | PubChem | |
URL | https://pubchem.ncbi.nlm.nih.gov | |
Description | Data deposited in or computed by PubChem | |
Molecular Weight |
246.26 g/mol | |
Details | Computed by PubChem 2.1 (PubChem release 2021.05.07) | |
Source | PubChem | |
URL | https://pubchem.ncbi.nlm.nih.gov | |
Description | Data deposited in or computed by PubChem | |
Retrosynthesis Analysis
AI-Powered Synthesis Planning: Our tool employs the Template_relevance Pistachio, Template_relevance Bkms_metabolic, Template_relevance Pistachio_ringbreaker, Template_relevance Reaxys, Template_relevance Reaxys_biocatalysis model, leveraging a vast database of chemical reactions to predict feasible synthetic routes.
One-Step Synthesis Focus: Specifically designed for one-step synthesis, it provides concise and direct routes for your target compounds, streamlining the synthesis process.
Accurate Predictions: Utilizing the extensive PISTACHIO, BKMS_METABOLIC, PISTACHIO_RINGBREAKER, REAXYS, REAXYS_BIOCATALYSIS database, our tool offers high-accuracy predictions, reflecting the latest in chemical research and data.
Strategy Settings
Precursor scoring | Relevance Heuristic |
---|---|
Min. plausibility | 0.01 |
Model | Template_relevance |
Template Set | Pistachio/Bkms_metabolic/Pistachio_ringbreaker/Reaxys/Reaxys_biocatalysis |
Top-N result to add to graph | 6 |
Feasible Synthetic Routes
Q1: What is the traditional use of Eriosema chinense Vogel and what compound contributes to its medicinal properties?
A1: The roots of Eriosema chinense Vogel are traditionally used by tribal communities in Meghalaya, India to treat diarrhea. [] Research suggests that Eriosematin E, a flavonoid present in the plant, is a key contributor to its antidiarrheal properties. [, , ]
Q2: How effective is Eriosematin E against different types of diarrhea-causing Escherichia coli?
A2: In silico studies using molecular docking simulations suggest that Eriosematin E shows varying degrees of effectiveness against different diarrhoeagenic E. coli strains. It exhibits the highest efficacy against enteropathogenic E. coli (EPEC), followed by enteroaggregative E. coli (EAEC) and enterotoxigenic E. coli (ETEC). [] Moderate effects were observed against uropathogenic E. coli (UPEC) and enterohaemorrhagic E. coli (EHEC). []
Q3: What is the mechanism of action of Eriosematin E in treating diarrhea?
A3: While the exact mechanism is still under investigation, research suggests that Eriosematin E may exert its antidiarrheal effects through multiple pathways:
- Inhibition of Intestinal Secretion: Eriosematin E might reduce fluid secretion in the intestines, a common characteristic of diarrhea. []
- Reduction of Nitric Oxide Production: Excessive nitric oxide contributes to diarrhea. Eriosematin E might help regulate nitric oxide levels in the gut. []
- Reactivation of Na+/K+-ATPase: This enzyme is crucial for maintaining electrolyte balance in the gut. Eriosematin E might help restore its activity, potentially alleviating diarrhea. []
Q4: What are the potential benefits of Eriosematin E in treating EPEC-induced diarrhea?
A4: Studies demonstrate that Eriosematin E can effectively reduce the severity of EPEC-induced diarrhea. It achieves this by: []
Q5: Are there any analytical methods used to quantify Eriosematin E?
A5: Yes, researchers have successfully used High-Performance Liquid Chromatography (HPLC) to standardize extracts of Eriosema chinense Vogel and quantify their Eriosematin E content. []
Avertissement et informations sur les produits de recherche in vitro
Veuillez noter que tous les articles et informations sur les produits présentés sur BenchChem sont destinés uniquement à des fins informatives. Les produits disponibles à l'achat sur BenchChem sont spécifiquement conçus pour des études in vitro, qui sont réalisées en dehors des organismes vivants. Les études in vitro, dérivées du terme latin "in verre", impliquent des expériences réalisées dans des environnements de laboratoire contrôlés à l'aide de cellules ou de tissus. Il est important de noter que ces produits ne sont pas classés comme médicaments et n'ont pas reçu l'approbation de la FDA pour la prévention, le traitement ou la guérison de toute condition médicale, affection ou maladie. Nous devons souligner que toute forme d'introduction corporelle de ces produits chez les humains ou les animaux est strictement interdite par la loi. Il est essentiel de respecter ces directives pour assurer la conformité aux normes légales et éthiques en matière de recherche et d'expérimentation.