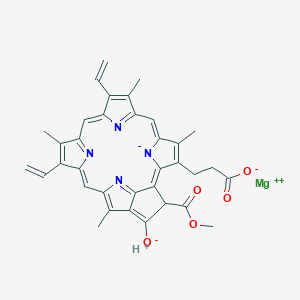
Divinyl protochlorophyllide
Vue d'ensemble
Description
Divinyl protochlorophyllide is a chlorophyll precursor found in photosynthetic organisms. It is a tetrapyrrole compound with a magnesium ion at its center and a fifth isocyclic pentanone ring. This compound plays a crucial role in the biosynthesis of chlorophyll, which is essential for photosynthesis in plants, algae, and cyanobacteria .
Méthodes De Préparation
Synthetic Routes and Reaction Conditions: Divinyl protochlorophyllide can be synthesized from protochlorophyllide a or chlorophyll. The synthesis involves the reduction of protochlorophyllide a to this compound using specific enzymes such as this compound a 8-vinyl reductase . The reaction conditions typically require a controlled environment with specific pH and temperature to ensure the proper functioning of the enzymes.
Industrial Production Methods: Industrial production of this compound is not widely practiced due to its specific role in photosynthesis and the complexity of its synthesis. it can be produced in laboratory settings using recombinant cyclases from eukaryotic oxygenic phototrophs .
Analyse Des Réactions Chimiques
Types of Reactions: Divinyl protochlorophyllide undergoes several types of chemical reactions, including:
Reduction: The reduction of this compound to monovinyl protochlorophyllide by this compound a 8-vinyl reductase.
Oxidation: The oxidation of this compound to form various chlorophyll derivatives.
Common Reagents and Conditions:
Reduction Reagents: Enzymes such as this compound a 8-vinyl reductase.
Oxidation Reagents: Oxygen and specific oxidizing agents under controlled conditions.
Major Products:
Monovinyl Protochlorophyllide: Formed from the reduction of this compound.
Chlorophyll Derivatives: Formed from the oxidation of this compound.
Applications De Recherche Scientifique
Divinyl protochlorophyllide has several scientific research applications, including:
Photosynthesis Research: It is used to study the biosynthesis of chlorophyll and the mechanisms of photosynthesis in plants, algae, and cyanobacteria.
Biotechnology: Recombinant cyclases from eukaryotic oxygenic phototrophs are used to produce this compound for various biotechnological applications.
Mécanisme D'action
Divinyl protochlorophyllide exerts its effects through its role in the biosynthesis of chlorophyll. The compound is converted to monovinyl protochlorophyllide by this compound a 8-vinyl reductase, which is then further converted to chlorophyllide a by NADPH:protochlorophyllide oxidoreductase . The molecular targets and pathways involved include the enzymes and intermediates in the chlorophyll biosynthetic pathway .
Comparaison Avec Des Composés Similaires
Monovinyl Protochlorophyllide: A direct product of the reduction of divinyl protochlorophyllide.
Chlorophyllide a: A subsequent product in the chlorophyll biosynthetic pathway.
Chlorophyll c Derivatives: Modified forms of chlorophyll a with similar roles in photosynthesis.
Uniqueness: this compound is unique due to its specific role as a precursor in the chlorophyll biosynthetic pathway. Unlike other chlorophyll derivatives, it undergoes specific enzymatic reductions and oxidations that are crucial for the formation of functional chlorophyll molecules .
Activité Biologique
Divinyl protochlorophyllide (DV-Pchlide) is a crucial intermediate in the biosynthesis of chlorophyll, primarily found in higher plants and certain cyanobacteria. Its biological activity is significant in various physiological processes, including photosynthesis, plant development, and potential therapeutic applications. This article delves into the biological activity of DV-Pchlide, supported by data tables, case studies, and detailed research findings.
1. Overview of this compound
This compound is characterized by its unique structure, featuring two vinyl groups at positions 3 and 8 of the tetrapyrrole ring. It serves as a precursor to chlorophyll a and b through enzymatic transformations involving divinyl reductase (DVR) and other enzymes in the chlorophyll biosynthetic pathway. The conversion of DV-Pchlide to monovinyl protochlorophyllide (MV-Pchlide) is essential for the formation of functional chlorophyll molecules that are vital for photosynthesis.
2. Enzymatic Conversion and Biosynthesis
Enzymatic Pathway:
The biosynthesis of chlorophyll from DV-Pchlide involves several key enzymes:
- Divinyl Reductase (DVR): This enzyme catalyzes the reduction of the 8-vinyl group to an ethyl group, facilitating the conversion of DV-Pchlide to MV-Pchlide .
- Protochlorophyllide Oxidoreductase (POR): In light-dependent reactions, POR reduces DV-Pchlide to chlorophyllide a, which is then converted to chlorophyll a .
Table 1: Key Enzymes in Chlorophyll Biosynthesis
Enzyme | Function | Substrate |
---|---|---|
Divinyl Reductase (DVR) | Reduces 8-vinyl group to ethyl | This compound |
Protochlorophyllide Oxidoreductase (POR) | Converts protochlorophyllide to chlorophyllide | Protochlorophyllide |
3.1 Photosynthetic Efficiency
DV-Pchlide plays a pivotal role in enhancing photosynthetic efficiency by serving as a precursor for chlorophyll synthesis. Studies indicate that plants with higher levels of DV-Pchlide exhibit improved growth under low-light conditions due to increased chlorophyll production .
3.2 Stress Response Mechanism
Research has shown that DV-Pchlide accumulation can be influenced by environmental stressors such as light intensity and nutrient availability. For instance, etiolated seedlings display varying levels of DV-Pchlide depending on their exposure to light, indicating its role in adaptive responses .
4. Case Studies
Case Study 1: Arabidopsis thaliana Mutants
A study involving Arabidopsis thaliana mutants revealed that those with defective DVR genes accumulated high levels of DV-Pchlide instead of MV-Pchlide. These mutants demonstrated altered growth patterns and photosynthetic capabilities compared to wild-type plants .
Case Study 2: Cyanobacterial Adaptations
In cyanobacteria such as Prochlorococcus marinus, the absence of DVR genes suggests an evolutionary adaptation where divinyl chlorophylls are utilized for efficient light absorption in oligotrophic ocean environments .
5. Therapeutic Potential
Recent studies have explored the potential therapeutic applications of chlorophyll derivatives, including DV-Pchlide. Research indicates that chlorophyllides exhibit antimicrobial and antitumor properties, making them candidates for developing new therapeutic agents against various diseases .
Propriétés
IUPAC Name |
magnesium;3-[11,16-bis(ethenyl)-3-methoxycarbonyl-12,17,21,26-tetramethyl-4-oxido-7,24,25-triaza-23-azanidahexacyclo[18.2.1.15,8.110,13.115,18.02,6]hexacosa-1,4,6,8(26),9,11,13(25),14,16,18(24),19,21-dodecaen-22-yl]propanoate;hydron | |
---|---|---|
Source | PubChem | |
URL | https://pubchem.ncbi.nlm.nih.gov | |
Description | Data deposited in or computed by PubChem | |
InChI |
InChI=1S/C35H32N4O5.Mg/c1-8-19-15(3)22-12-24-17(5)21(10-11-28(40)41)32(38-24)30-31(35(43)44-7)34(42)29-18(6)25(39-33(29)30)14-27-20(9-2)16(4)23(37-27)13-26(19)36-22;/h8-9,12-14,31H,1-2,10-11H2,3-7H3,(H3,36,37,38,39,40,41,42);/q;+2/p-2 | |
Source | PubChem | |
URL | https://pubchem.ncbi.nlm.nih.gov | |
Description | Data deposited in or computed by PubChem | |
InChI Key |
YXBIPIDDNARELO-UHFFFAOYSA-L | |
Source | PubChem | |
URL | https://pubchem.ncbi.nlm.nih.gov | |
Description | Data deposited in or computed by PubChem | |
Canonical SMILES |
[H+].CC1=C(C2=C3C(C(=C4C3=NC(=C4C)C=C5C(=C(C(=N5)C=C6C(=C(C(=N6)C=C1[N-]2)C)C=C)C)C=C)[O-])C(=O)OC)CCC(=O)[O-].[Mg+2] | |
Source | PubChem | |
URL | https://pubchem.ncbi.nlm.nih.gov | |
Description | Data deposited in or computed by PubChem | |
Molecular Formula |
C35H30MgN4O5 | |
Source | PubChem | |
URL | https://pubchem.ncbi.nlm.nih.gov | |
Description | Data deposited in or computed by PubChem | |
Molecular Weight |
610.9 g/mol | |
Source | PubChem | |
URL | https://pubchem.ncbi.nlm.nih.gov | |
Description | Data deposited in or computed by PubChem | |
CAS No. |
18433-30-2 | |
Record name | 2,4-Divinylprotochlorophyllide | |
Source | ChemIDplus | |
URL | https://pubchem.ncbi.nlm.nih.gov/substance/?source=chemidplus&sourceid=0018433302 | |
Description | ChemIDplus is a free, web search system that provides access to the structure and nomenclature authority files used for the identification of chemical substances cited in National Library of Medicine (NLM) databases, including the TOXNET system. | |
Retrosynthesis Analysis
AI-Powered Synthesis Planning: Our tool employs the Template_relevance Pistachio, Template_relevance Bkms_metabolic, Template_relevance Pistachio_ringbreaker, Template_relevance Reaxys, Template_relevance Reaxys_biocatalysis model, leveraging a vast database of chemical reactions to predict feasible synthetic routes.
One-Step Synthesis Focus: Specifically designed for one-step synthesis, it provides concise and direct routes for your target compounds, streamlining the synthesis process.
Accurate Predictions: Utilizing the extensive PISTACHIO, BKMS_METABOLIC, PISTACHIO_RINGBREAKER, REAXYS, REAXYS_BIOCATALYSIS database, our tool offers high-accuracy predictions, reflecting the latest in chemical research and data.
Strategy Settings
Precursor scoring | Relevance Heuristic |
---|---|
Min. plausibility | 0.01 |
Model | Template_relevance |
Template Set | Pistachio/Bkms_metabolic/Pistachio_ringbreaker/Reaxys/Reaxys_biocatalysis |
Top-N result to add to graph | 6 |
Feasible Synthetic Routes
Avertissement et informations sur les produits de recherche in vitro
Veuillez noter que tous les articles et informations sur les produits présentés sur BenchChem sont destinés uniquement à des fins informatives. Les produits disponibles à l'achat sur BenchChem sont spécifiquement conçus pour des études in vitro, qui sont réalisées en dehors des organismes vivants. Les études in vitro, dérivées du terme latin "in verre", impliquent des expériences réalisées dans des environnements de laboratoire contrôlés à l'aide de cellules ou de tissus. Il est important de noter que ces produits ne sont pas classés comme médicaments et n'ont pas reçu l'approbation de la FDA pour la prévention, le traitement ou la guérison de toute condition médicale, affection ou maladie. Nous devons souligner que toute forme d'introduction corporelle de ces produits chez les humains ou les animaux est strictement interdite par la loi. Il est essentiel de respecter ces directives pour assurer la conformité aux normes légales et éthiques en matière de recherche et d'expérimentation.