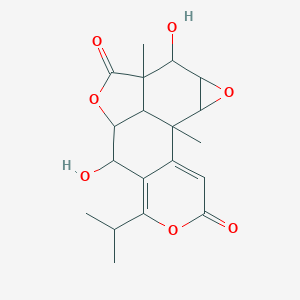
Nagilactone C
Vue d'ensemble
Description
La nagilactone est un groupe de lactones terpénoïdes bioactives initialement isolées de la plante Podocarpus nagi au Japon à la fin des années 1960 . Ces composés sont des produits naturels tétracycliques qui présentent une variété d'effets pharmacologiques, notamment des activités antifongiques, anti-athéroscléreuses, anti-inflammatoires et anticancéreuses . Les dérivés les plus actifs, tels que les nagilactones C, E et F, présentent de puissantes activités anticancéreuses contre différentes lignées de cellules cancéreuses et modèles tumoraux .
Applications De Recherche Scientifique
Nagilactones have a wide range of scientific research applications. They are used in chemistry, biology, medicine, and industry due to their diverse biological activities . Some of the notable applications include:
Anticancer Activity: Nagilactones, particularly nagilactones C, E, and F, exhibit potent anticancer activities against various cancer cell lines and tumor models.
Antifungal Activity: These compounds display antifungal properties, making them useful in developing antifungal agents.
Anti-inflammatory Activity: Nagilactones have anti-inflammatory effects, which can be utilized in treating inflammatory diseases.
Anti-atherosclerosis Activity: These compounds also show potential in preventing or treating atherosclerosis.
Mécanisme D'action
Target of Action
Nagilactone C, a tetracyclic natural product isolated from various Podocarpus species, has been found to target eukaryotic ribosomes and different protein kinases . The primary targets of this compound include protein kinases such as RIOK2 and JAK2 . These targets play a crucial role in cell proliferation and survival, making them important targets for anticancer therapies .
Mode of Action
This compound interacts with its targets by binding to eukaryotic ribosomes, inhibiting protein synthesis . It also inhibits different protein kinases, such as RIOK2 and JAK2 . This interaction results in a drug-induced inhibition of cell proliferation, coupled with a cell cycle perturbation and induction of apoptosis .
Biochemical Pathways
The anticancer activity of this compound mainly derives from three complementary actions . Firstly, it induces inhibition of cell proliferation coupled with a cell cycle perturbation and induction of apoptosis . Secondly, it blocks the epithelial to mesenchymal cell transition, contributing to an inhibition of cancer cell migration and invasion . Lastly, it has the capacity to modulate the PD-L1 immune checkpoint . Different molecular effectors have been implicated in the antitumor activity, chiefly the AP-1 pathway blocked upon activation of the JNK/c-Jun axis .
Pharmacokinetics
Its potent anticancer activities against different cancer cell lines and tumor models suggest that it may have favorable bioavailability .
Result of Action
The result of this compound’s action is a significant reduction in cell proliferation and induction of apoptosis in cancer cells . It also inhibits cancer cell migration and invasion by blocking the epithelial to mesenchymal cell transition . Furthermore, it modulates the PD-L1 immune checkpoint, potentially enhancing the immune response against cancer cells .
Action Environment
As a natural product isolated from various podocarpus species, it is likely that factors such as temperature, ph, and presence of other bioactive compounds could potentially influence its action .
Safety and Hazards
Safety measures include avoiding dust formation, breathing mist, gas or vapours, and contacting with skin and eye. Use of personal protective equipment and chemical impermeable gloves is recommended. Ensure adequate ventilation, remove all sources of ignition, evacuate personnel to safe areas, and keep people away from and upwind of spill/leak .
Analyse Biochimique
Biochemical Properties
Nagilactone C interacts with several enzymes and proteins in biochemical reactions. It is a potent inhibitor of protein synthesis, binding to eukaryotic ribosomes . Additionally, it has been postulated that this compound inhibits different protein kinases, such as RIOK2 and JAK2 .
Cellular Effects
This compound has potent antiproliferative activity against human fibrosarcoma and murine colon carcinoma tumor cell lines . It exerts its effects by inhibiting cell proliferation, perturbing the cell cycle, and inducing apoptosis . Furthermore, this compound can block the epithelial to mesenchymal cell transition, contributing to an inhibition of cancer cell migration and invasion .
Molecular Mechanism
The anticancer activity of this compound mainly derives from three complementary actions: drug-induced inhibition of cell proliferation, blockade of the epithelial to mesenchymal cell transition, and modulation of the PD-L1 immune checkpoint . The AP-1 pathway, blocked upon activation of the JNK/c-Jun axis, has been implicated as a key molecular effector in the antitumor activity of this compound .
Dosage Effects in Animal Models
The initial report showed a dose-dependent activity of this compound against the P-388 leukemia in mice, with the drug injected daily at 10–20–40 mg/kg intraperitoneally .
Metabolic Pathways
Given its role as a potent inhibitor of protein synthesis and its interactions with various protein kinases, it is likely that this compound influences several metabolic pathways .
Subcellular Localization
Given its role as a potent inhibitor of protein synthesis, it is likely that this compound interacts with ribosomes, which are located in the cytoplasm of the cell .
Méthodes De Préparation
Voies de synthèse et conditions de réaction
La nagilactone F peut être synthétisée par oxydation avec du tétraacétate de plomb dans le benzène sous irradiation UV . La réaction se déroule par oxydation allylique par le tétraacétate de plomb, et le cation allylique résultant est piégé par le groupe acide carboxylique pour former la partie lactone D-cyclique .
Méthodes de production industrielle
Les méthodes de production industrielle des nagilactones impliquent généralement l'extraction des graines, des racines, des rameaux, des écorces ou des feuilles de diverses espèces de Podocarpus . Des méthodes chromatographiques complètes sont utilisées pour isoler différentes nagilactones, telles que la nagilactone B, la nagilactone N3 et la 2-épinagilactone B .
Analyse Des Réactions Chimiques
Types de réactions
Les nagilactones subissent diverses réactions chimiques, notamment l'oxydation, la réduction et la substitution . Par exemple, la nagilactone F est obtenue par oxydation avec du tétraacétate de plomb .
Réactifs et conditions usuels
Les réactifs courants utilisés dans la synthèse des nagilactones comprennent le tétraacétate de plomb pour les réactions d'oxydation . Les conditions de réaction impliquent souvent une irradiation UV et l'utilisation de solvants tels que le benzène .
Principaux produits formés
Les principaux produits formés à partir de ces réactions comprennent différents dérivés de nagilactones, tels que la nagilactone F, qui est formée par oxydation allylique .
Applications de la recherche scientifique
Les nagilactones ont un large éventail d'applications de recherche scientifique. Elles sont utilisées en chimie, en biologie, en médecine et dans l'industrie en raison de leurs diverses activités biologiques . Certaines des applications notables incluent :
Activité anticancéreuse : Les nagilactones, en particulier les nagilactones C, E et F, présentent de puissantes activités anticancéreuses contre diverses lignées de cellules cancéreuses et modèles tumoraux.
Activité antifongique : Ces composés présentent des propriétés antifongiques, ce qui les rend utiles dans le développement d'agents antifongiques.
Activité anti-inflammatoire : Les nagilactones ont des effets anti-inflammatoires, qui peuvent être utilisés dans le traitement des maladies inflammatoires.
Activité anti-athéroscléreuse : Ces composés montrent également un potentiel dans la prévention ou le traitement de l'athérosclérose.
Mécanisme d'action
Le mécanisme d'action des nagilactones implique plusieurs actions complémentaires :
Inhibition de la prolifération cellulaire : Les nagilactones induisent une inhibition de la prolifération cellulaire couplée à une perturbation du cycle cellulaire et une induction de l'apoptose.
Blocage de la transition épithéliale vers mésenchymateuse : Elles bloquent la transition cellulaire épithéliale vers mésenchymateuse, contribuant à l'inhibition de la migration et de l'invasion des cellules cancéreuses.
Modulation du point de contrôle immunitaire PD-L1 : Les nagilactones ont la capacité de moduler le point de contrôle immunitaire PD-L1.
Différents effecteurs moléculaires sont impliqués dans l'activité antitumorale des nagilactones, principalement la voie AP-1 bloquée lors de l'activation de l'axe JNK/c-Jun . La nagilactone C est un puissant inhibiteur de la synthèse protéique se liant aux ribosomes eucaryotes, tandis que la nagilactone E inhibe différentes protéines kinases, telles que RIOK2 et JAK2 .
Comparaison Avec Des Composés Similaires
Les nagilactones sont structurellement apparentées à d'autres composés isolés de plantes Podocarpus, tels que les podolactones, les oidiolactones et les inumakilactones . Ces composés partagent des activités biologiques similaires mais diffèrent dans leurs structures spécifiques et leurs mécanismes d'action . Par exemple :
Podolactones : Ce sont des nor- ou des dinor-diterpénoïdes contenant une γ-lactone entre les carbones 6–19 et une δ-lactone entre les carbones 12–14.
Oidiolactones : Ces composés ont montré des effets inhibiteurs prometteurs contre Candida albicans et d'autres champignons.
Inumakilactones : Il s'agit d'un autre groupe de terpènes présentant des structures et des activités biologiques uniques.
Les nagilactones sont uniques en raison de leurs puissantes activités anticancéreuses et de leur capacité à moduler le point de contrôle immunitaire PD-L1, ce qui n'est pas communément observé dans d'autres composés similaires .
Propriétés
IUPAC Name |
5,10-dihydroxy-1,6-dimethyl-12-propan-2-yl-3,8,13-trioxapentacyclo[7.7.1.02,4.06,17.011,16]heptadeca-11,15-diene-7,14-dione | |
---|---|---|
Details | Computed by Lexichem TK 2.7.0 (PubChem release 2021.05.07) | |
Source | PubChem | |
URL | https://pubchem.ncbi.nlm.nih.gov | |
Description | Data deposited in or computed by PubChem | |
InChI |
InChI=1S/C19H22O7/c1-6(2)11-9-7(5-8(20)24-11)18(3)14-12(10(9)21)26-17(23)19(14,4)15(22)13-16(18)25-13/h5-6,10,12-16,21-22H,1-4H3 | |
Details | Computed by InChI 1.0.6 (PubChem release 2021.05.07) | |
Source | PubChem | |
URL | https://pubchem.ncbi.nlm.nih.gov | |
Description | Data deposited in or computed by PubChem | |
InChI Key |
DGNOPGIIPQKNHD-UHFFFAOYSA-N | |
Details | Computed by InChI 1.0.6 (PubChem release 2021.05.07) | |
Source | PubChem | |
URL | https://pubchem.ncbi.nlm.nih.gov | |
Description | Data deposited in or computed by PubChem | |
Canonical SMILES |
CC(C)C1=C2C(C3C4C(C(C5C(C4(C2=CC(=O)O1)C)O5)O)(C(=O)O3)C)O | |
Details | Computed by OEChem 2.3.0 (PubChem release 2021.05.07) | |
Source | PubChem | |
URL | https://pubchem.ncbi.nlm.nih.gov | |
Description | Data deposited in or computed by PubChem | |
Molecular Formula |
C19H22O7 | |
Details | Computed by PubChem 2.1 (PubChem release 2021.05.07) | |
Source | PubChem | |
URL | https://pubchem.ncbi.nlm.nih.gov | |
Description | Data deposited in or computed by PubChem | |
Molecular Weight |
362.4 g/mol | |
Details | Computed by PubChem 2.1 (PubChem release 2021.05.07) | |
Source | PubChem | |
URL | https://pubchem.ncbi.nlm.nih.gov | |
Description | Data deposited in or computed by PubChem | |
CAS No. |
24338-53-2 | |
Record name | Nagilactone C | |
Source | DTP/NCI | |
URL | https://dtp.cancer.gov/dtpstandard/servlet/dwindex?searchtype=NSC&outputformat=html&searchlist=211500 | |
Description | The NCI Development Therapeutics Program (DTP) provides services and resources to the academic and private-sector research communities worldwide to facilitate the discovery and development of new cancer therapeutic agents. | |
Explanation | Unless otherwise indicated, all text within NCI products is free of copyright and may be reused without our permission. Credit the National Cancer Institute as the source. | |
Retrosynthesis Analysis
AI-Powered Synthesis Planning: Our tool employs the Template_relevance Pistachio, Template_relevance Bkms_metabolic, Template_relevance Pistachio_ringbreaker, Template_relevance Reaxys, Template_relevance Reaxys_biocatalysis model, leveraging a vast database of chemical reactions to predict feasible synthetic routes.
One-Step Synthesis Focus: Specifically designed for one-step synthesis, it provides concise and direct routes for your target compounds, streamlining the synthesis process.
Accurate Predictions: Utilizing the extensive PISTACHIO, BKMS_METABOLIC, PISTACHIO_RINGBREAKER, REAXYS, REAXYS_BIOCATALYSIS database, our tool offers high-accuracy predictions, reflecting the latest in chemical research and data.
Strategy Settings
Precursor scoring | Relevance Heuristic |
---|---|
Min. plausibility | 0.01 |
Model | Template_relevance |
Template Set | Pistachio/Bkms_metabolic/Pistachio_ringbreaker/Reaxys/Reaxys_biocatalysis |
Top-N result to add to graph | 6 |
Feasible Synthetic Routes
Q1: What is Nagilactone C and where is it found?
A1: this compound is a norditerpene dilactone primarily isolated from various species of Podocarpus, a type of coniferous tree. [, , ]
Q2: What are the known biological activities of this compound?
A2: Research indicates that this compound exhibits insecticidal activity against various insects like housefly larvae, light-brown apple moth, and codling moth. [, ] It also demonstrates antiproliferative activity against human and murine tumor cell lines, suggesting potential anticancer properties. [, ] Furthermore, studies have identified this compound as a potent inhibitor of Dengue virus infection. []
Q3: How does this compound exert its insecticidal effect?
A3: While the exact mechanism is not fully elucidated in the provided research, studies show that this compound is toxic to housefly larvae when ingested, affecting larval growth, pupation, and adult emergence. [] It also shows insecticidal activity against other insect species, suggesting a broader mechanism of action. [, ]
Q4: How potent is this compound as an antiproliferative agent?
A4: this compound exhibits potent antiproliferative activity against human fibrosarcoma (HT-1080) and murine colon carcinoma (Colon 26-L5) cell lines with ED50 values of 2.3 and 1.2 μg/mL, respectively. []
Q5: How does the structure of this compound contribute to its biological activities?
A6: While detailed structure-activity relationship (SAR) studies are not described within the provided research, the presence of the lactone groups and the overall norditerpene skeleton are likely crucial for its biological activities. [] Further research exploring modifications to its structure would provide valuable insights into SAR.
Q6: Has this compound's interaction with specific biological targets been studied?
A7: Yes, research indicates that this compound targets the eukaryotic translation apparatus, specifically affecting translation elongation. [] Additionally, a study elucidated the crystal structure of this compound bound to the yeast 80S ribosome. [] This binding interaction provides insights into its mechanism as a protein synthesis inhibitor.
Q7: What are the implications of this compound's interaction with the ribosome?
A8: The binding of this compound to the ribosome and subsequent inhibition of eukaryotic protein synthesis likely contributes to its various biological activities, including its anticancer, antiviral, and insecticidal effects. [, ] Disrupting protein synthesis can have significant downstream effects on cellular processes and viral replication.
Q8: Are there any known instances of resistance to this compound?
A8: While the provided research does not explicitly mention resistance mechanisms, it is plausible that prolonged exposure to this compound could lead to the development of resistance in target organisms, as seen with other bioactive compounds.
Q9: What is the current state of research on this compound?
A9: Current research primarily focuses on understanding this compound's mechanism of action against various targets, exploring its potential therapeutic applications (anticancer, antiviral, insecticidal), and investigating its biosynthesis in Podocarpus species.
Q10: Are there any analytical techniques used to identify and quantify this compound?
A11: Researchers commonly employ spectroscopic techniques like Nuclear Magnetic Resonance (NMR), Infrared Spectroscopy (IR), and high-resolution mass spectrometry (HR-ESI-MS) to identify and characterize this compound. [, ] These techniques provide detailed structural information and can confirm the compound's identity.
Avertissement et informations sur les produits de recherche in vitro
Veuillez noter que tous les articles et informations sur les produits présentés sur BenchChem sont destinés uniquement à des fins informatives. Les produits disponibles à l'achat sur BenchChem sont spécifiquement conçus pour des études in vitro, qui sont réalisées en dehors des organismes vivants. Les études in vitro, dérivées du terme latin "in verre", impliquent des expériences réalisées dans des environnements de laboratoire contrôlés à l'aide de cellules ou de tissus. Il est important de noter que ces produits ne sont pas classés comme médicaments et n'ont pas reçu l'approbation de la FDA pour la prévention, le traitement ou la guérison de toute condition médicale, affection ou maladie. Nous devons souligner que toute forme d'introduction corporelle de ces produits chez les humains ou les animaux est strictement interdite par la loi. Il est essentiel de respecter ces directives pour assurer la conformité aux normes légales et éthiques en matière de recherche et d'expérimentation.