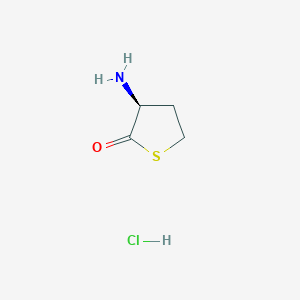
L-Homocysteine thiolactone hydrochloride
Vue d'ensemble
Description
La thiolactone de L-homocystéine (chlorhydrate) est un composé de formule chimique C4H7NOS · HCl et d'un poids moléculaire de 153,63 g/mol . Il s'agit d'un thioester cyclique à cinq chaînons de l'acide aminé homocystéine . Ce composé est généré à partir de l'homocystéine à la suite d'une réaction d'édition d'erreurs, principalement de la méthionyl-ARNt synthétase . La thiolactone de L-homocystéine (chlorhydrate) est connue pour son rôle dans la prévention de l'incorporation translationnelle de l'homocystéine dans les protéines .
Mécanisme D'action
Target of Action
L-Homocysteine thiolactone hydrochloride primarily targets plasma proteins . It binds to these proteins and induces conformational changes . It is also used to study post-translational modification of proteins, especially at lysine residues .
Mode of Action
The compound acts as an intramolecular thioester of Homocysteine . It prevents the translational incorporation of homocysteine into proteins . This interaction with its targets leads to changes in the structure and function of the proteins .
Biochemical Pathways
This compound is generated from homocysteine as a result of an error-editing reaction, principally, of methionyl-tRNA synthetase . It can form an activated derivative HcyAMP and then catalyzes an intramolecular thioester bond formation in a reaction between the side-chain—SH group and activated carbonyl group of Hcy, affording HTL .
Pharmacokinetics
It is known that the compound can induce elevated hhcy (hyperhomocysteinemia) in mice , suggesting that it is absorbed and distributed in the body to exert its effects.
Result of Action
The molecular and cellular effects of this compound’s action include the induction of elevated HHcy (hyperhomocysteinemia) in mice . It also shows a significant increase in pro-inflammatory cytokines IP-10, TNFα, IL-1β, and IL6 . Furthermore, it has been associated with detrimental effects on vascular function and may induce oxidative stress .
Méthodes De Préparation
Voies de synthèse et conditions de réaction
La thiolactone de L-homocystéine (chlorhydrate) peut être synthétisée à partir d'acides aminés homocystéine ou méthionine . La synthèse implique la formation d'un thioester cyclique à cinq chaînons par une réaction d'édition d'erreurs . Les conditions de réaction comprennent généralement l'utilisation de la méthionyl-ARNt synthétase pour catalyser la formation de la thiolactone .
Méthodes de production industrielle
Les méthodes de production industrielle de la thiolactone de L-homocystéine (chlorhydrate) ne sont pas largement documentées.
Analyse Des Réactions Chimiques
Types de réactions
La thiolactone de L-homocystéine (chlorhydrate) subit plusieurs types de réactions chimiques, notamment :
Oxydation : Le composé peut être oxydé pour former des disulfures.
Réduction : Les réactions de réduction peuvent convertir la thiolactone en homocystéine.
Substitution : Le cycle thiolactone peut être ouvert par des nucléophiles tels que les amines ou les groupes hydroxyle.
Réactifs et conditions courants
Les réactifs couramment utilisés dans ces réactions comprennent des agents oxydants pour les réactions d'oxydation, des agents réducteurs pour les réactions de réduction et des nucléophiles pour les réactions de substitution .
Principaux produits formés
Les principaux produits formés par ces réactions comprennent des disulfures à partir de l'oxydation, de l'homocystéine à partir de la réduction et divers amides ou esters à partir de réactions de substitution .
Applications de recherche scientifique
La thiolactone de L-homocystéine (chlorhydrate) a plusieurs applications de recherche scientifique, notamment :
Chimie : Elle est utilisée comme élément de base pour la synthèse organique et bio-organique.
Industrie : Elle est utilisée dans la science des polymères et le développement de matériaux durables.
Mécanisme d'action
La thiolactone de L-homocystéine (chlorhydrate) exerce ses effets en empêchant l'incorporation translationnelle de l'homocystéine dans les protéines . Cette modification peut entraîner des dommages aux protéines via un mécanisme radical thiyle, conduisant à l'agrégation des protéines et à la neurotoxicité .
Applications De Recherche Scientifique
L-Homocysteine thiolactone (hydrochloride) has several scientific research applications, including:
Comparaison Avec Des Composés Similaires
Composés similaires
Les composés similaires à la thiolactone de L-homocystéine (chlorhydrate) comprennent :
- Chlorhydrate de thiolactone de DL-homocystéine
- Acide L-homocystéique
- Chlorhydrate de L-homoarginine
- Chlorhydrate de L-cystéine
Unicité
La thiolactone de L-homocystéine (chlorhydrate) est unique en raison de son rôle dans la prévention de l'incorporation de l'homocystéine dans les protéines et de sa capacité à induire des modifications post-traductionnelles . Cela en fait un outil précieux pour l'étude des modifications des protéines et des maladies associées .
Activité Biologique
L-Homocysteine thiolactone hydrochloride (L-Hcy TLHC) is a chemically reactive metabolite of homocysteine, a sulfur-containing amino acid. This compound has garnered attention due to its significant biological activities and implications in cardiovascular health, oxidative stress, and potential therapeutic applications. This article provides a detailed overview of the biological activity of L-Hcy TLHC, supported by relevant research findings, data tables, and case studies.
Overview of this compound
L-Hcy TLHC is formed through the metabolic conversion of homocysteine and is known for its ability to acylate proteins, leading to post-translational modifications that can affect protein function. The thiolactone form is particularly reactive and has been implicated in various pathological processes, including cardiovascular diseases (CVD) and oxidative stress.
1. Cardiovascular Effects
Research indicates that L-Hcy TLHC has notable effects on cardiac function. A study utilizing isolated rat hearts demonstrated that administration of L-Hcy TLHC resulted in decreased cardiac contractility and coronary flow:
Parameter | Control (Mean ± SD) | L-Hcy TLHC (10 μM) (Mean ± SD) | P-Value |
---|---|---|---|
dp/dt max | 1230 ± 150 | 980 ± 120 | <0.05 |
dp/dt min | -850 ± 100 | -900 ± 110 | NS |
SLVP | 80 ± 10 | 60 ± 15 | <0.05 |
Coronary Flow (CF) | 15 ± 2 | 10 ± 1 | <0.05 |
The study highlighted that while L-Hcy TLHC decreased dp/dt max and coronary flow, it did not significantly alter oxidative stress markers such as hydrogen peroxide levels or superoxide anion concentrations when administered alone .
2. Oxidative Stress
L-Hcy TLHC has been shown to influence oxidative stress pathways. In combination with gasotransmitter inhibitors (e.g., L-NAME), it reduced oxidative stress markers, suggesting a complex interaction between homocysteine metabolism and oxidative stress mechanisms:
- Oxidative Stress Markers :
- TBARS: Thiobarbituric acid reactive substances
- NO2: Nitrite concentration
- O2−: Superoxide anion
- H2O2: Hydrogen peroxide concentration
The combination treatment led to significant reductions in these markers, indicating that L-Hcy TLHC may have a protective effect against oxidative damage under specific conditions .
3. Risk Marker for Cardiovascular Disease
A prospective study involving patients with suspected coronary artery disease found that urinary excretion levels of homocysteine thiolactone were significantly associated with the risk of acute myocardial infarction (AMI). The hazard ratio for AMI risk was reported as follows:
Urinary Hcy-Thiolactone/Creatinine Ratio | Hazard Ratio (HR) | Confidence Interval (CI) |
---|---|---|
Below Median | 1.58 | 1.10–2.26 |
Above Median | 2.72 | 1.47–5.03 |
This association remained significant even after adjusting for confounding factors such as age and gender, suggesting that elevated levels of urinary Hcy-thiolactone may serve as a sensitive biomarker for cardiovascular risk assessment .
Mechanistic Insights
The biological activity of L-Hcy TLHC is largely attributed to its ability to modify proteins through acylation, which can lead to altered protein function and contribute to pathological conditions such as endothelial dysfunction and vascular damage. The mechanism by which homocysteine induces these effects includes:
- Generation of reactive oxygen species (ROS)
- Impairment of nitric oxide bioavailability
- Induction of inflammatory pathways
These processes are critical in understanding how elevated homocysteine levels contribute to cardiovascular diseases .
Case Study: Impact on Cardiac Function
In an experimental model using isolated rat hearts, researchers observed that the administration of L-Hcy TLHC led to significant changes in cardiac parameters compared to controls. The study concluded that the thiolactone form of homocysteine plays a detrimental role in cardiac contractility and coronary flow regulation.
Case Study: Urinary Biomarker for AMI Risk
A large cohort study evaluated urinary excretion levels of Hcy-thiolactone among patients with confirmed coronary artery disease. The findings indicated a strong correlation between elevated urinary Hcy-thiolactone levels and increased incidence of AMI, highlighting its potential as a predictive biomarker .
Propriétés
IUPAC Name |
(3S)-3-aminothiolan-2-one;hydrochloride | |
---|---|---|
Source | PubChem | |
URL | https://pubchem.ncbi.nlm.nih.gov | |
Description | Data deposited in or computed by PubChem | |
InChI |
InChI=1S/C4H7NOS.ClH/c5-3-1-2-7-4(3)6;/h3H,1-2,5H2;1H/t3-;/m0./s1 | |
Source | PubChem | |
URL | https://pubchem.ncbi.nlm.nih.gov | |
Description | Data deposited in or computed by PubChem | |
InChI Key |
ZSEGSUBKDDEALH-DFWYDOINSA-N | |
Source | PubChem | |
URL | https://pubchem.ncbi.nlm.nih.gov | |
Description | Data deposited in or computed by PubChem | |
Canonical SMILES |
C1CSC(=O)C1N.Cl | |
Source | PubChem | |
URL | https://pubchem.ncbi.nlm.nih.gov | |
Description | Data deposited in or computed by PubChem | |
Isomeric SMILES |
C1CSC(=O)[C@H]1N.Cl | |
Source | PubChem | |
URL | https://pubchem.ncbi.nlm.nih.gov | |
Description | Data deposited in or computed by PubChem | |
Molecular Formula |
C4H8ClNOS | |
Source | PubChem | |
URL | https://pubchem.ncbi.nlm.nih.gov | |
Description | Data deposited in or computed by PubChem | |
DSSTOX Substance ID |
DTXSID60953745 | |
Record name | 3-Aminothiolan-2-one--hydrogen chloride (1/1) | |
Source | EPA DSSTox | |
URL | https://comptox.epa.gov/dashboard/DTXSID60953745 | |
Description | DSSTox provides a high quality public chemistry resource for supporting improved predictive toxicology. | |
Molecular Weight |
153.63 g/mol | |
Source | PubChem | |
URL | https://pubchem.ncbi.nlm.nih.gov | |
Description | Data deposited in or computed by PubChem | |
CAS No. |
31828-68-9 | |
Record name | L-Homocysteine thiolactone hydrochloride | |
Source | CAS Common Chemistry | |
URL | https://commonchemistry.cas.org/detail?cas_rn=31828-68-9 | |
Description | CAS Common Chemistry is an open community resource for accessing chemical information. Nearly 500,000 chemical substances from CAS REGISTRY cover areas of community interest, including common and frequently regulated chemicals, and those relevant to high school and undergraduate chemistry classes. This chemical information, curated by our expert scientists, is provided in alignment with our mission as a division of the American Chemical Society. | |
Explanation | The data from CAS Common Chemistry is provided under a CC-BY-NC 4.0 license, unless otherwise stated. | |
Record name | Homocysteine thiolactone hydrochloride, L- | |
Source | ChemIDplus | |
URL | https://pubchem.ncbi.nlm.nih.gov/substance/?source=chemidplus&sourceid=0031828689 | |
Description | ChemIDplus is a free, web search system that provides access to the structure and nomenclature authority files used for the identification of chemical substances cited in National Library of Medicine (NLM) databases, including the TOXNET system. | |
Record name | 3-Aminothiolan-2-one--hydrogen chloride (1/1) | |
Source | EPA DSSTox | |
URL | https://comptox.epa.gov/dashboard/DTXSID60953745 | |
Description | DSSTox provides a high quality public chemistry resource for supporting improved predictive toxicology. | |
Record name | (S)-3-aminodihydrothiophen-2(3H)-one hydrochloride | |
Source | European Chemicals Agency (ECHA) | |
URL | https://echa.europa.eu/substance-information/-/substanceinfo/100.046.189 | |
Description | The European Chemicals Agency (ECHA) is an agency of the European Union which is the driving force among regulatory authorities in implementing the EU's groundbreaking chemicals legislation for the benefit of human health and the environment as well as for innovation and competitiveness. | |
Explanation | Use of the information, documents and data from the ECHA website is subject to the terms and conditions of this Legal Notice, and subject to other binding limitations provided for under applicable law, the information, documents and data made available on the ECHA website may be reproduced, distributed and/or used, totally or in part, for non-commercial purposes provided that ECHA is acknowledged as the source: "Source: European Chemicals Agency, http://echa.europa.eu/". Such acknowledgement must be included in each copy of the material. ECHA permits and encourages organisations and individuals to create links to the ECHA website under the following cumulative conditions: Links can only be made to webpages that provide a link to the Legal Notice page. | |
Record name | HOMOCYSTEINE THIOLACTONE HYDROCHLORIDE, L- | |
Source | FDA Global Substance Registration System (GSRS) | |
URL | https://gsrs.ncats.nih.gov/ginas/app/beta/substances/2HTL9Q12FE | |
Description | The FDA Global Substance Registration System (GSRS) enables the efficient and accurate exchange of information on what substances are in regulated products. Instead of relying on names, which vary across regulatory domains, countries, and regions, the GSRS knowledge base makes it possible for substances to be defined by standardized, scientific descriptions. | |
Explanation | Unless otherwise noted, the contents of the FDA website (www.fda.gov), both text and graphics, are not copyrighted. They are in the public domain and may be republished, reprinted and otherwise used freely by anyone without the need to obtain permission from FDA. Credit to the U.S. Food and Drug Administration as the source is appreciated but not required. | |
Retrosynthesis Analysis
AI-Powered Synthesis Planning: Our tool employs the Template_relevance Pistachio, Template_relevance Bkms_metabolic, Template_relevance Pistachio_ringbreaker, Template_relevance Reaxys, Template_relevance Reaxys_biocatalysis model, leveraging a vast database of chemical reactions to predict feasible synthetic routes.
One-Step Synthesis Focus: Specifically designed for one-step synthesis, it provides concise and direct routes for your target compounds, streamlining the synthesis process.
Accurate Predictions: Utilizing the extensive PISTACHIO, BKMS_METABOLIC, PISTACHIO_RINGBREAKER, REAXYS, REAXYS_BIOCATALYSIS database, our tool offers high-accuracy predictions, reflecting the latest in chemical research and data.
Strategy Settings
Precursor scoring | Relevance Heuristic |
---|---|
Min. plausibility | 0.01 |
Model | Template_relevance |
Template Set | Pistachio/Bkms_metabolic/Pistachio_ringbreaker/Reaxys/Reaxys_biocatalysis |
Top-N result to add to graph | 6 |
Feasible Synthetic Routes
Q1: What is the role of L-Homocysteine thiolactone hydrochloride in the synthesis of radiotracers for Positron Emission Tomography (PET)?
A1: this compound serves as a precursor in the synthesis of (S-[¹¹C]-methyl)-L-methionine (¹¹C-MET), a radiotracer commonly used in PET imaging. The process involves an on-column [¹¹C]methylation reaction with [¹¹C]methyl iodide ([¹¹C]CH3I) using this compound as the starting material [, ]. This reaction efficiently yields ¹¹C-MET with high radiochemical and enantiomeric purity suitable for preclinical and clinical PET imaging studies.
Q2: How does the stabilization of Filamentous actin (F-actin) impact the effects of this compound on endothelial cells?
A2: Research suggests that stabilizing F-actin in EA.hy926 endothelial cells can mitigate some of the negative effects associated with this compound exposure []. Specifically, F-actin stabilization appears to protect against L-Homocysteine-induced reductions in cell mobility and may enhance cell-cell junction integrity by increasing the expression of junctional β-catenin and influencing the localization of Zonula occludens-1 (ZO-1).
Q3: Are there alternative methylation agents to [¹¹C]methyl iodide for producing ¹¹C-labeled radiotracers like ¹¹C-MET?
A3: Yes, research indicates that [¹¹C]methyl bromide ([¹¹C]CH3Br) can serve as an effective alternative methylation agent for synthesizing ¹¹C-labeled radiotracers []. This method, utilizing a one-pot wet method on a commercial [¹¹C]CH3I synthesizer, successfully produced ¹¹C-MET with high radiochemical purity and yield comparable to traditional methods. This alternative approach offers a potentially simpler and more efficient route for producing ¹¹C-labeled tracers for clinical PET imaging.
Q4: Have any polymorphic forms of this compound been identified?
A4: Yes, crystallographic studies have revealed the existence of two distinct polymorphic forms of this compound, along with a hybrid form []. The discovery of these polymorphic forms highlights the structural complexity of this compound and may have implications for its stability, solubility, and overall behavior in different environments.
Avertissement et informations sur les produits de recherche in vitro
Veuillez noter que tous les articles et informations sur les produits présentés sur BenchChem sont destinés uniquement à des fins informatives. Les produits disponibles à l'achat sur BenchChem sont spécifiquement conçus pour des études in vitro, qui sont réalisées en dehors des organismes vivants. Les études in vitro, dérivées du terme latin "in verre", impliquent des expériences réalisées dans des environnements de laboratoire contrôlés à l'aide de cellules ou de tissus. Il est important de noter que ces produits ne sont pas classés comme médicaments et n'ont pas reçu l'approbation de la FDA pour la prévention, le traitement ou la guérison de toute condition médicale, affection ou maladie. Nous devons souligner que toute forme d'introduction corporelle de ces produits chez les humains ou les animaux est strictement interdite par la loi. Il est essentiel de respecter ces directives pour assurer la conformité aux normes légales et éthiques en matière de recherche et d'expérimentation.