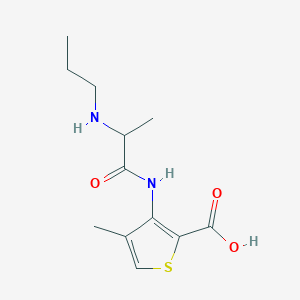
Acide articainique
Vue d'ensemble
Description
Articainic acid is a metabolite of articaine, a widely used local anesthetic in dentistry. Articaine is unique among amide local anesthetics due to its thiophene ring, which enhances its lipid solubility and potency. Articainic acid is formed through the hydrolysis of articaine in the blood, making it an inactive metabolite that is excreted by the kidneys .
Applications De Recherche Scientifique
Pharmacokinetics of Articainic Acid
Articainic acid is primarily formed through the hydrolysis of articaine, an amide local anesthetic. The pharmacokinetic profile of articainic acid is crucial for understanding its effectiveness and safety in clinical applications.
- Absorption and Elimination : After administration, articaine is rapidly absorbed and metabolized into articainic acid. Studies have shown that the elimination half-life of articainic acid ranges from approximately 0.90 to 2.44 hours, depending on the method of administration and individual patient factors .
- Renal Excretion : A significant portion (40-70%) of the administered dose is excreted as articainic acid, indicating its role as a primary metabolite . The renal clearance rates for articainic acid vary widely, reflecting individual metabolic differences.
Clinical Applications
Articainic acid has been studied extensively for its applications in local anesthesia, particularly in dental procedures.
- Efficacy in Dental Procedures : Clinical studies have compared the efficacy of articaine (and by extension, its metabolite) to other local anesthetics such as lidocaine. Articaine has demonstrated comparable effectiveness with a rapid onset of action, making it suitable for various dental procedures .
- Regional Anesthesia : Articaine's ability to be metabolized into articainic acid during regional anesthesia has been documented. For instance, a study involving intravenous regional anesthesia showed that after tourniquet release, plasma concentrations of articainic acid were higher than those of articaine itself, indicating significant metabolism during the procedure .
Case Studies
Several case studies highlight the practical applications and implications of articainic acid in clinical settings:
- Intravenous Regional Anesthesia :
-
Pharmacokinetics in Red Deer :
- Research on red deer indicated that following subcutaneous administration around antler pedicles, articainic acid was detected at significant concentrations shortly after administration. This suggests potential applications in veterinary medicine for pain management during procedures like antler removal .
- Comparative Studies :
Data Tables
The following tables summarize key pharmacokinetic parameters and clinical findings related to articainic acid.
Parameter | Articaine | Articainic Acid |
---|---|---|
Elimination Half-Life | 0.54 - 2.44 hours | 0.90 - 2.44 hours |
Renal Excretion | 40-70% as metabolite | Major pathway |
Maximum Plasma Concentration (Cmax) | Varies by study | Detected post-administration |
Mécanisme D'action
Target of Action
Articainic acid, also known as Articaine, is primarily used as a local anesthetic in dentistry . Its primary targets are the nerve fibers responsible for pain sensation. By acting on these targets, Articaine effectively blocks the transmission of nerve impulses, leading to a temporary loss of sensation in the area where it is applied .
Mode of Action
Articaine works by blocking both the initiation and conduction of nerve impulses. It achieves this by increasing the threshold for electrical excitation in the nerve, slowing the propagation of the nerve impulse, and reducing the rate of rise of the action potential . This effectively prevents the sensation of pain from being transmitted to the brain.
Biochemical Pathways
Articaine is unique among amide local anesthetics in that it contains a thiophene ring, which allows for greater lipid solubility and potency. This means a greater portion of an administered dose can enter neurons . It is also the only amide anesthetic containing an ester group, which allows for hydrolysis by unspecific blood esterases .
Pharmacokinetics
Articaine is rapidly metabolized in the blood by esterases into its inactive metabolite, articainic acid . Approximately 90% of Articaine undergoes this rapid hydrolysis, with the resulting articainic acid then excreted by the kidneys . The elimination half-time of articaine is about 20 minutes . The rapid breakdown of articaine to articainic acid contributes to its very low systemic toxicity, allowing for the possibility of repeated injections .
Result of Action
The primary result of Articaine’s action is the successful induction of local anesthesia. This is achieved through its action on nerve fibers, which blocks the transmission of pain signals. Articaine has been found to have a higher likelihood of achieving anesthetic success than other local anesthetics like lidocaine .
Action Environment
The efficacy and safety of Articaine have been demonstrated in various environments, including dental procedures and ambulatory spinal anesthesia . Environmental factors such as the presence of vasoactive agents like epinephrine can influence Articaine’s action. For instance, epinephrine can reduce the systemic uptake of local anesthetics, resulting in a longer duration of action and a lower peak concentration .
Analyse Biochimique
Biochemical Properties
Articainic acid is produced through the hydrolysis of articaine, facilitated by serum esterases . This process begins immediately after the administration of articaine . The presence of a thiophene ring in articaine, instead of a benzene ring, allows a greater portion of the administered dose to enter neurons, contributing to its potency .
Cellular Effects
The primary role of articainic acid in cells is as a metabolite of articaine. It is currently unclear whether articainic acid itself has significant direct effects on cellular processes. It has been noted that articainic acid had no effect on EEG, ECG, blood pressure, and heart rate in one study .
Molecular Mechanism
Articainic acid is the result of the hydrolysis of articaine, a process that begins immediately after the administration of articaine . This hydrolysis is facilitated by serum esterases
Temporal Effects in Laboratory Settings
The metabolism of articaine to articainic acid is a rapid process, with about 90% of articaine quickly metabolized via hydrolysis in the blood into articainic acid . This rapid breakdown is related to a very low systemic toxicity, allowing for the possibility of repeated injections .
Metabolic Pathways
Articainic acid is primarily formed through the hydrolysis of articaine, facilitated by serum esterases . This metabolic pathway is unique to articaine among amide local anesthetics due to its ester group .
Transport and Distribution
Articainic acid is the product of the metabolism of articaine in the blood . It is excreted by the kidney in the form of articainic acid glucuronide
Méthodes De Préparation
Articainic acid is primarily produced as a metabolite of articaine. The synthesis of articaine involves the following steps:
Synthesis of 2-thiophenecarboxylic acid: This is achieved through the Friedel-Crafts acylation of thiophene.
Formation of 4-methyl-3-(2-propylamino)-propionamido-2-thiophenecarboxylic acid: This involves the reaction of 2-thiophenecarboxylic acid with propylamine and subsequent acylation.
Esterification: The final step involves the esterification of the carboxylic acid group to form articaine.
Industrial production of articainic acid involves the hydrolysis of articaine in the presence of esterases, which are enzymes that catalyze the hydrolysis of ester bonds .
Analyse Des Réactions Chimiques
Articainic acid undergoes several chemical reactions, including:
Hydrolysis: Articaine is hydrolyzed in the blood to form articainic acid.
Glucuronidation: Articainic acid is further metabolized in the liver to form articainic acid glucuronide, which is excreted by the kidneys.
Common reagents and conditions used in these reactions include esterases for hydrolysis and glucuronosyltransferases for glucuronidation. The major products formed from these reactions are articainic acid and articainic acid glucuronide .
Comparaison Avec Des Composés Similaires
Articainic acid is unique among local anesthetic metabolites due to its rapid hydrolysis and excretion. Similar compounds include:
Lidocaine: Metabolized in the liver to form monoethylglycinexylidide and glycinexylidide.
Prilocaine: Metabolized to orthotoluidine, which can cause methemoglobinemia.
Bupivacaine: Metabolized in the liver to form pipecolylxylidine.
Articainic acid’s rapid hydrolysis and excretion make it less likely to accumulate in the body, reducing the risk of toxicity compared to other local anesthetic metabolites .
Activité Biologique
Articainic acid is a key metabolite of articaine, a widely used local anesthetic in dental and medical procedures. Understanding the biological activity of articainic acid is essential for assessing its pharmacological effects, safety profile, and potential therapeutic applications. This article reviews the available literature on the biological activity of articainic acid, including its pharmacokinetics, metabolic pathways, and case studies that illustrate its clinical relevance.
1. Pharmacokinetics of Articainic Acid
Articainic acid is primarily formed through the hydrolysis of articaine, which is metabolized by plasma cholinesterases. The pharmacokinetic properties of articainic acid have been studied in various contexts, including human patients and animal models.
Key Findings:
- Elimination Half-Life : The elimination half-life of articainic acid ranges from approximately 0.90 hours to 1.12 hours depending on the study and the method of administration .
- Plasma Concentrations : After intravenous administration of articaine, peak plasma concentrations (C_max) of articainic acid were observed at around 0.5 hours post-administration, with values reaching approximately 762.6 ng/mL in controlled studies .
- Excretion : About 75% of articainic acid is excreted unchanged by the kidneys, while the remainder undergoes glucuronidation before elimination .
2. Metabolic Pathways
Articainic acid's metabolism involves several pathways that influence its biological activity and safety:
- Hydrolysis : Articaine is hydrolyzed to articainic acid by plasma cholinesterases shortly after administration. This rapid metabolism contributes to its short duration of action.
- Glucuronidation : In the kidneys, articainic acid can undergo further metabolism to form glucuronide conjugates, which are then excreted in urine .
3. Biological Activity and Toxicity
Although articainic acid is considered an inactive metabolite compared to other local anesthetics' metabolites, its role in potential toxicity has been examined:
- Neurotoxicity : Unlike some metabolites of lidocaine that may exhibit neurotoxic effects, articainic acid does not demonstrate significant neurotoxicity . This characteristic makes articaine a safer option for local anesthesia.
- Clinical Observations : Reports indicate that elevated levels of articainic acid can occur in patients with renal impairment, raising concerns about local anesthetic systemic toxicity (LAST) under certain conditions .
4. Case Studies
Several case studies highlight the clinical implications of articainic acid's pharmacokinetics and biological activity:
- Study on Regional Anesthesia : In a study involving ten patients undergoing hand surgery with intravenous regional anesthesia using articaine, it was noted that after tourniquet release, concentrations of articainic acid exceeded those of articaine itself, indicating significant regional metabolism during the procedure .
- Animal Studies : Research conducted on red deer demonstrated a clear pharmacokinetic profile for both articaine and its metabolite, allowing for insights into their absorption and elimination characteristics in veterinary applications .
5. Summary Table of Key Data
Parameter | Articaine | Articainic Acid |
---|---|---|
Molecular Structure | Amide local anesthetic | Inactive metabolite |
Elimination Half-Life | ~1 hour | ~0.90 - 1.12 hours |
C_max (Plasma Concentration) | Varies by study | ~762.6 ng/mL |
Excretion | Primarily renal | Unchanged (~75%) |
Clinical Significance | Effective local anesthesia; low neurotoxicity | Minimal clinical activity |
Propriétés
IUPAC Name |
4-methyl-3-[2-(propylamino)propanoylamino]thiophene-2-carboxylic acid | |
---|---|---|
Source | PubChem | |
URL | https://pubchem.ncbi.nlm.nih.gov | |
Description | Data deposited in or computed by PubChem | |
InChI |
InChI=1S/C12H18N2O3S/c1-4-5-13-8(3)11(15)14-9-7(2)6-18-10(9)12(16)17/h6,8,13H,4-5H2,1-3H3,(H,14,15)(H,16,17) | |
Source | PubChem | |
URL | https://pubchem.ncbi.nlm.nih.gov | |
Description | Data deposited in or computed by PubChem | |
InChI Key |
LUPKINKNYDFRIB-UHFFFAOYSA-N | |
Source | PubChem | |
URL | https://pubchem.ncbi.nlm.nih.gov | |
Description | Data deposited in or computed by PubChem | |
Canonical SMILES |
CCCNC(C)C(=O)NC1=C(SC=C1C)C(=O)O | |
Source | PubChem | |
URL | https://pubchem.ncbi.nlm.nih.gov | |
Description | Data deposited in or computed by PubChem | |
Molecular Formula |
C12H18N2O3S | |
Source | PubChem | |
URL | https://pubchem.ncbi.nlm.nih.gov | |
Description | Data deposited in or computed by PubChem | |
DSSTOX Substance ID |
DTXSID50921296 | |
Record name | 3-{[1-Hydroxy-2-(propylamino)propylidene]amino}-4-methylthiophene-2-carboxylic acid | |
Source | EPA DSSTox | |
URL | https://comptox.epa.gov/dashboard/DTXSID50921296 | |
Description | DSSTox provides a high quality public chemistry resource for supporting improved predictive toxicology. | |
Molecular Weight |
270.35 g/mol | |
Source | PubChem | |
URL | https://pubchem.ncbi.nlm.nih.gov | |
Description | Data deposited in or computed by PubChem | |
CAS No. |
114176-52-2 | |
Record name | 2-Carboxyarticaine | |
Source | ChemIDplus | |
URL | https://pubchem.ncbi.nlm.nih.gov/substance/?source=chemidplus&sourceid=0114176522 | |
Description | ChemIDplus is a free, web search system that provides access to the structure and nomenclature authority files used for the identification of chemical substances cited in National Library of Medicine (NLM) databases, including the TOXNET system. | |
Record name | 3-{[1-Hydroxy-2-(propylamino)propylidene]amino}-4-methylthiophene-2-carboxylic acid | |
Source | EPA DSSTox | |
URL | https://comptox.epa.gov/dashboard/DTXSID50921296 | |
Description | DSSTox provides a high quality public chemistry resource for supporting improved predictive toxicology. | |
Record name | ARTICAINE ACID | |
Source | FDA Global Substance Registration System (GSRS) | |
URL | https://gsrs.ncats.nih.gov/ginas/app/beta/substances/99R4WP7QC7 | |
Description | The FDA Global Substance Registration System (GSRS) enables the efficient and accurate exchange of information on what substances are in regulated products. Instead of relying on names, which vary across regulatory domains, countries, and regions, the GSRS knowledge base makes it possible for substances to be defined by standardized, scientific descriptions. | |
Explanation | Unless otherwise noted, the contents of the FDA website (www.fda.gov), both text and graphics, are not copyrighted. They are in the public domain and may be republished, reprinted and otherwise used freely by anyone without the need to obtain permission from FDA. Credit to the U.S. Food and Drug Administration as the source is appreciated but not required. | |
Retrosynthesis Analysis
AI-Powered Synthesis Planning: Our tool employs the Template_relevance Pistachio, Template_relevance Bkms_metabolic, Template_relevance Pistachio_ringbreaker, Template_relevance Reaxys, Template_relevance Reaxys_biocatalysis model, leveraging a vast database of chemical reactions to predict feasible synthetic routes.
One-Step Synthesis Focus: Specifically designed for one-step synthesis, it provides concise and direct routes for your target compounds, streamlining the synthesis process.
Accurate Predictions: Utilizing the extensive PISTACHIO, BKMS_METABOLIC, PISTACHIO_RINGBREAKER, REAXYS, REAXYS_BIOCATALYSIS database, our tool offers high-accuracy predictions, reflecting the latest in chemical research and data.
Strategy Settings
Precursor scoring | Relevance Heuristic |
---|---|
Min. plausibility | 0.01 |
Model | Template_relevance |
Template Set | Pistachio/Bkms_metabolic/Pistachio_ringbreaker/Reaxys/Reaxys_biocatalysis |
Top-N result to add to graph | 6 |
Feasible Synthetic Routes
Avertissement et informations sur les produits de recherche in vitro
Veuillez noter que tous les articles et informations sur les produits présentés sur BenchChem sont destinés uniquement à des fins informatives. Les produits disponibles à l'achat sur BenchChem sont spécifiquement conçus pour des études in vitro, qui sont réalisées en dehors des organismes vivants. Les études in vitro, dérivées du terme latin "in verre", impliquent des expériences réalisées dans des environnements de laboratoire contrôlés à l'aide de cellules ou de tissus. Il est important de noter que ces produits ne sont pas classés comme médicaments et n'ont pas reçu l'approbation de la FDA pour la prévention, le traitement ou la guérison de toute condition médicale, affection ou maladie. Nous devons souligner que toute forme d'introduction corporelle de ces produits chez les humains ou les animaux est strictement interdite par la loi. Il est essentiel de respecter ces directives pour assurer la conformité aux normes légales et éthiques en matière de recherche et d'expérimentation.