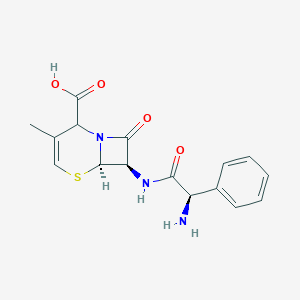
Impureté F de céphalexine
Vue d'ensemble
Description
Δ2-Cephalexin is an isomeric impurity of the semi-synthetic antibiotic Cephalexin
Applications De Recherche Scientifique
Contrôle de la qualité pharmaceutique
L'impureté F de céphalexine est utilisée dans l'évaluation du profil de dégradation de la céphalexine (CEF) dans les formes de capsules pharmaceutiques . Cela implique d'exposer des échantillons de la substance pharmaceutique active et du produit fini correspondant à diverses conditions de stress, telles que l'hydrolyse acide, l'hydrolyse basique, l'oxydation, le traitement thermique, le traitement photolytique et l'humidité contrôlée . La génération de produits de dégradation potentiels, y compris l'this compound, est évaluée .
Développement de méthodes d'indication de stabilité
L'étude de l'this compound contribue au développement d'une méthode d'indication de stabilité utilisant la chromatographie liquide à ultra haute pression couplée à la détection par matrice de diodes et à la spectrométrie de masse à haute résolution . Cette méthode fournit des informations sur les voies de dégradation possibles et la détection de composés inconnus .
Profil d'impuretés
L'this compound est utilisée dans l'évaluation multidimensionnelle des profils d'impuretés pour les antibiotiques génériques de céphalexine . La chromatographie liquide à haute performance (HPLC) avec élution en gradient suivie d'une détection par matrice de photodiodes ou par spectrométrie de masse fournit des informations précieuses concernant la nature des impuretés observées .
Études de dégradation des médicaments
La céphalexine et l'impureté F diffèrent par la position de la double liaison du cycle dihydrothiazine . Tous les principaux fragments de céphalexine pourraient être identifiés dans les données de produits de dégradation, ce qui permet de déduire que l'impureté RRT_1,05 était l'impureté F
Mécanisme D'action
Target of Action
Cephalexin Impurity F, also known as DELTA2-CEPHALEXIN, is a derivative of the first-generation cephalosporin antibiotic, Cephalexin . The primary targets of this compound are the bacterial cell walls, specifically the peptidoglycan layer, which provides mechanical stability to the cell .
Mode of Action
Cephalexin Impurity F, like its parent compound Cephalexin, contains a beta-lactam and a dihydrothiazide . It inhibits bacterial cell wall synthesis, leading to the breakdown and eventual death of the bacterial cell . Unlike penicillins, cephalosporins are more resistant to the action of beta-lactamase , an enzyme produced by certain bacteria that can inactivate beta-lactam antibiotics.
Biochemical Pathways
This binding inhibits the cross-linking of peptidoglycan chains necessary for bacterial cell wall strength and rigidity .
Pharmacokinetics
The human jejunal permeability (Peff) of Cephalexin is 1.56 x 10^-4 cm/s, indicating its importance in drug absorption .
Result of Action
The result of the action of Cephalexin Impurity F is the inhibition of bacterial cell wall synthesis, leading to cell lysis and death . This makes it effective against a broad range of gram-positive and gram-negative bacteria.
Action Environment
The action of Cephalexin Impurity F can be influenced by various environmental factors. For instance, it has been found that Cephalexin is generally stable when exposed to temperatures up to 60 °C, ultraviolet light or visible light in the 300–800 nm wavelength range, and 75% relative humidity . Under acidic, basic, hydrogen peroxide, and metal ion oxidative conditions, cephalexin showed degradation susceptibility . These findings suggest that similar environmental factors could potentially influence the action, efficacy, and stability of Cephalexin Impurity F.
Analyse Biochimique
Biochemical Properties
It is known that it differs from cephalexin in the position of the double bond of the dihydrothiazine ring . This structural difference could potentially influence its interactions with enzymes, proteins, and other biomolecules.
Cellular Effects
Given its structural similarity to cephalexin, it may have similar effects on cell function, including impacts on cell signaling pathways, gene expression, and cellular metabolism .
Molecular Mechanism
Like cephalexin, it may interact with biomolecules, inhibit or activate enzymes, and cause changes in gene expression .
Temporal Effects in Laboratory Settings
In laboratory settings, Cephalexin Impurity F has been observed under forced degradation conditions . It was found that cephalexin is generally stable when exposed to temperatures up to 60 °C, ultraviolet light, or visible light in the 300–800 nm wavelength range and 75% relative humidity . Under acidic, basic, hydrogen peroxide, and metal ion oxidative conditions, cephalexin showed degradation susceptibility, as demonstrated by the decrease in content and the formation of degradation products .
Metabolic Pathways
Given its structural similarity to cephalexin, it may be involved in similar metabolic pathways .
Propriétés
IUPAC Name |
(6R,7R)-7-[[(2R)-2-amino-2-phenylacetyl]amino]-3-methyl-8-oxo-5-thia-1-azabicyclo[4.2.0]oct-3-ene-2-carboxylic acid | |
---|---|---|
Source | PubChem | |
URL | https://pubchem.ncbi.nlm.nih.gov | |
Description | Data deposited in or computed by PubChem | |
InChI |
InChI=1S/C16H17N3O4S/c1-8-7-24-15-11(14(21)19(15)12(8)16(22)23)18-13(20)10(17)9-5-3-2-4-6-9/h2-7,10-12,15H,17H2,1H3,(H,18,20)(H,22,23)/t10-,11-,12?,15-/m1/s1 | |
Source | PubChem | |
URL | https://pubchem.ncbi.nlm.nih.gov | |
Description | Data deposited in or computed by PubChem | |
InChI Key |
UALOAEFJDPITFY-XOGJBXBPSA-N | |
Source | PubChem | |
URL | https://pubchem.ncbi.nlm.nih.gov | |
Description | Data deposited in or computed by PubChem | |
Canonical SMILES |
CC1=CSC2C(C(=O)N2C1C(=O)O)NC(=O)C(C3=CC=CC=C3)N | |
Source | PubChem | |
URL | https://pubchem.ncbi.nlm.nih.gov | |
Description | Data deposited in or computed by PubChem | |
Isomeric SMILES |
CC1=CS[C@@H]2[C@@H](C(=O)N2C1C(=O)O)NC(=O)[C@@H](C3=CC=CC=C3)N | |
Source | PubChem | |
URL | https://pubchem.ncbi.nlm.nih.gov | |
Description | Data deposited in or computed by PubChem | |
Molecular Formula |
C16H17N3O4S | |
Source | PubChem | |
URL | https://pubchem.ncbi.nlm.nih.gov | |
Description | Data deposited in or computed by PubChem | |
DSSTOX Substance ID |
DTXSID80554692 | |
Record name | (6R,7R)-7-{[(2R)-2-Amino-2-phenylacetyl]amino}-3-methyl-8-oxo-5-thia-1-azabicyclo[4.2.0]oct-3-ene-2-carboxylic acid | |
Source | EPA DSSTox | |
URL | https://comptox.epa.gov/dashboard/DTXSID80554692 | |
Description | DSSTox provides a high quality public chemistry resource for supporting improved predictive toxicology. | |
Molecular Weight |
347.4 g/mol | |
Source | PubChem | |
URL | https://pubchem.ncbi.nlm.nih.gov | |
Description | Data deposited in or computed by PubChem | |
CAS No. |
79750-46-2 | |
Record name | (6R,7R)-7-{[(2R)-2-Amino-2-phenylacetyl]amino}-3-methyl-8-oxo-5-thia-1-azabicyclo[4.2.0]oct-3-ene-2-carboxylic acid | |
Source | EPA DSSTox | |
URL | https://comptox.epa.gov/dashboard/DTXSID80554692 | |
Description | DSSTox provides a high quality public chemistry resource for supporting improved predictive toxicology. | |
Retrosynthesis Analysis
AI-Powered Synthesis Planning: Our tool employs the Template_relevance Pistachio, Template_relevance Bkms_metabolic, Template_relevance Pistachio_ringbreaker, Template_relevance Reaxys, Template_relevance Reaxys_biocatalysis model, leveraging a vast database of chemical reactions to predict feasible synthetic routes.
One-Step Synthesis Focus: Specifically designed for one-step synthesis, it provides concise and direct routes for your target compounds, streamlining the synthesis process.
Accurate Predictions: Utilizing the extensive PISTACHIO, BKMS_METABOLIC, PISTACHIO_RINGBREAKER, REAXYS, REAXYS_BIOCATALYSIS database, our tool offers high-accuracy predictions, reflecting the latest in chemical research and data.
Strategy Settings
Precursor scoring | Relevance Heuristic |
---|---|
Min. plausibility | 0.01 |
Model | Template_relevance |
Template Set | Pistachio/Bkms_metabolic/Pistachio_ringbreaker/Reaxys/Reaxys_biocatalysis |
Top-N result to add to graph | 6 |
Feasible Synthetic Routes
Avertissement et informations sur les produits de recherche in vitro
Veuillez noter que tous les articles et informations sur les produits présentés sur BenchChem sont destinés uniquement à des fins informatives. Les produits disponibles à l'achat sur BenchChem sont spécifiquement conçus pour des études in vitro, qui sont réalisées en dehors des organismes vivants. Les études in vitro, dérivées du terme latin "in verre", impliquent des expériences réalisées dans des environnements de laboratoire contrôlés à l'aide de cellules ou de tissus. Il est important de noter que ces produits ne sont pas classés comme médicaments et n'ont pas reçu l'approbation de la FDA pour la prévention, le traitement ou la guérison de toute condition médicale, affection ou maladie. Nous devons souligner que toute forme d'introduction corporelle de ces produits chez les humains ou les animaux est strictement interdite par la loi. Il est essentiel de respecter ces directives pour assurer la conformité aux normes légales et éthiques en matière de recherche et d'expérimentation.