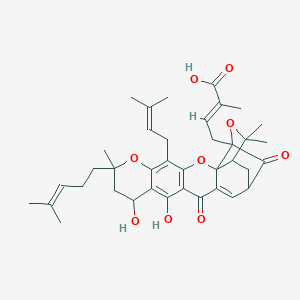
Acide néogambogique
Vue d'ensemble
Description
L'acide néo-gambogique, également connu sous le nom d'acide gambogique, est un composé naturel présent dans la résine des espèces de Garcinia, en particulier Garcinia hanburyi. Ce composé a suscité un intérêt considérable en raison de ses puissantes propriétés anticancéreuses. L'acide néo-gambogique est une xanthone polyprenylée, une classe de composés connus pour leurs diverses activités biologiques, notamment des effets anti-inflammatoires, antibactériens et anticancéreux .
Applications De Recherche Scientifique
Anticancer Properties
Neogambogic acid has been extensively studied for its potential as an anticancer agent. Research indicates that it can inhibit the proliferation of cancer cells and induce apoptosis through various mechanisms.
Mechanisms of Action:
- Induction of Apoptosis: Neogambogic acid promotes apoptosis in cancer cells by activating the Fas/FasL pathway and increasing the expression of pro-apoptotic proteins such as Bax while decreasing anti-apoptotic proteins like Bcl-2. This was demonstrated in human breast cancer MCF-7 cells, where treatment with neogambogic acid resulted in a significant reduction in cell viability and an increase in apoptotic cell populations .
- Cell Cycle Arrest: Neogambogic acid induces G0/G1 phase arrest, thereby inhibiting cell cycle progression. This effect was observed in multiple cancer cell lines, including Ehrlich ascites carcinoma and Lewis lung carcinoma models .
Case Study: MCF-7 Cells
- Experimental Design: MCF-7 cells were treated with varying concentrations of neogambogic acid. The results showed a dose-dependent decrease in cell proliferation and an increase in apoptotic markers.
- Results Summary:
Cardioprotective Effects
Recent studies have highlighted the cardioprotective effects of neogambogic acid, particularly in models of sepsis-induced myocardial injury.
Mechanisms of Action:
- Reduction of Cardiac Apoptosis: Neogambogic acid has been shown to decrease myocardial apoptosis by modulating apoptotic pathways, evidenced by reduced expression of cleaved caspase-3 and increased levels of Bcl-2 in septic mice .
- Anti-inflammatory Effects: The compound reduces inflammation markers such as IL-1β, IL-6, and TNF-α in cardiac tissues, contributing to improved cardiac function during septic conditions .
Case Study: Septic Mice
- Experimental Design: Mice subjected to lipopolysaccharide (LPS) treatment were administered neogambogic acid.
- Results Summary:
Antibacterial Activity
Neogambogic acid exhibits significant antibacterial properties, particularly against Methicillin-resistant Staphylococcus aureus (MRSA).
Mechanisms of Action:
- Inhibition of Biofilm Formation: Studies have shown that neogambogic acid can effectively inhibit both planktonic growth and biofilm formation of MRSA strains. This is crucial given the challenges posed by antibiotic-resistant bacteria .
Case Study: MRSA Inhibition
- Experimental Design: In vitro assays were conducted to evaluate the effectiveness of neogambogic acid against MRSA.
- Results Summary:
Comparative Data Table
Analyse Biochimique
Biochemical Properties
Neogambogic acid has been found to interact with undecaprenyl diphosphate synthase (UPPS), an essential enzyme required for bacterial cell wall formation . It significantly inhibits the activity of UPPS in a dose-dependent manner . This interaction is characterized by neogambogic acid occupying the substrate binding pocket of UPPS, preventing the natural substrates from entering .
Cellular Effects
Neogambogic acid has been shown to have significant inhibitory activity toward MRSA . It not only effectively inhibits planktonic MRSA strains in vivo and in vitro, but also has strong inhibitory effects on MRSA biofilms formation . In addition, it has been found to suppress the viability and spheroid formation ability of colorectal cancer (CRC) cells .
Molecular Mechanism
Neogambogic acid exerts its effects at the molecular level through various mechanisms. It has been found to reduce the expression of S. aureus virulence factors by inhibiting the saeRS two-component system . This results in the inhibition of MRSA invasion to the host . Furthermore, it induces apoptosis in human MCF-7 cells .
Temporal Effects in Laboratory Settings
While specific studies detailing the temporal effects of neogambogic acid in laboratory settings are limited, its inhibitory effects on MRSA and human MCF-7 cells have been observed in both in vivo and in vitro studies .
Dosage Effects in Animal Models
The dosage effects of neogambogic acid in animal models are not well-documented. It has been demonstrated that neogambogic acid can improve E. faecalis-induced undesirable inflammation in a mouse infection model .
Metabolic Pathways
It has been found to inhibit the activity of UPPS, an enzyme involved in the synthesis of bacterial cell walls .
Transport and Distribution
It has been found to occupy the substrate binding pocket of UPPS, suggesting that it may be transported to the site of this enzyme .
Subcellular Localization
Given its interaction with UPPS, an enzyme located in the bacterial cell membrane, it may be localized to this region in bacterial cells .
Méthodes De Préparation
Voies synthétiques et conditions de réaction : La synthèse de l'acide néo-gambogique implique plusieurs étapes, à partir de l'extraction de la résine des espèces de Garcinia. La résine est ensuite soumise à divers processus de purification pour isoler l'acide néo-gambogique. La voie synthétique implique généralement l'utilisation de solvants organiques et de techniques chromatographiques pour atteindre une pureté élevée .
Méthodes de production industrielle : La production industrielle de l'acide néo-gambogique en est encore à ses débuts. Les progrès des méthodes biotechnologiques, telles que la modification biosynthétique, sont explorés pour améliorer le rendement et la pureté de l'acide néo-gambogique. Ces méthodes impliquent l'utilisation de micro-organismes génétiquement modifiés pour produire le composé en grandes quantités .
Analyse Des Réactions Chimiques
Types de réactions : L'acide néo-gambogique subit diverses réactions chimiques, notamment l'oxydation, la réduction et la substitution. Ces réactions sont essentielles pour modifier le composé afin d'améliorer son activité biologique et de réduire sa toxicité .
Réactifs et conditions courants :
Oxydation : Des agents oxydants courants tels que le permanganate de potassium et le peroxyde d'hydrogène sont utilisés.
Réduction : Des agents réducteurs comme le borohydrure de sodium et l'hydrure de lithium et d'aluminium sont utilisés.
Substitution : Les réactions d'halogénation utilisant des réactifs comme le brome et le chlore sont courantes.
Principaux produits : Les principaux produits formés à partir de ces réactions comprennent divers dérivés de l'acide néo-gambogique, qui sont étudiés pour leurs propriétés anticancéreuses améliorées et leurs effets secondaires réduits .
4. Applications de la recherche scientifique
L'acide néo-gambogique a un large éventail d'applications de recherche scientifique :
Chimie : Utilisé comme précurseur pour synthétiser de nouveaux composés aux avantages thérapeutiques potentiels.
Médecine : Enquête sur son potentiel comme agent anticancéreux, en particulier pour le traitement du cancer du sein, du cancer du poumon et du glioblastome
5. Mécanisme d'action
L'acide néo-gambogique exerce ses effets principalement par l'induction de l'apoptose dans les cellules cancéreuses. Il active la voie Fas/FasL, conduisant à l'activation des caspases, qui sont essentielles au processus apoptotique. De plus, l'acide néo-gambogique inhibe la voie de signalisation Akt, entraînant un stress oxydatif mitochondrial et la mort cellulaire . Le composé cible également la synthétase de diphosphate d'undecaprénol, une enzyme essentielle à la formation de la paroi cellulaire bactérienne, présentant ainsi des propriétés antibactériennes .
Composés similaires :
Acide gambogique : Une autre xanthone polyprenylée présente dans les espèces de Garcinia, connue pour ses propriétés anticancéreuses.
Mangostine : Un dérivé de la xanthone doté de puissantes activités antioxydantes et anticancéreuses.
Unicité : L'acide néo-gambogique est unique en raison de ses propriétés anticancéreuses et antibactériennes doubles. Sa capacité à induire l'apoptose par le biais de multiples voies et son efficacité contre un large éventail de lignées de cellules cancéreuses en font un candidat prometteur pour de futures recherches et développements .
Mécanisme D'action
Neo-gambogic acid exerts its effects primarily through the induction of apoptosis in cancer cells. It activates the Fas/FasL pathway, leading to the activation of caspases, which are crucial for the apoptotic process. Additionally, neo-gambogic acid inhibits the Akt signaling pathway, resulting in mitochondrial oxidative stress and cell death . The compound also targets undecaprenyl diphosphate synthase, an enzyme essential for bacterial cell wall formation, thereby exhibiting antibacterial properties .
Comparaison Avec Des Composés Similaires
Gambogic Acid: Another polyprenylated xanthone found in Garcinia species, known for its anticancer properties.
Mangostin: A xanthone derivative with potent antioxidant and anticancer activities.
Uniqueness: Neo-gambogic acid is unique due to its dual anticancer and antibacterial properties. Its ability to induce apoptosis through multiple pathways and its effectiveness against a wide range of cancer cell lines make it a promising candidate for further research and development .
Activité Biologique
Neogambogic acid (NGA), a derivative of gambogic acid, has garnered attention for its diverse biological activities, particularly in the fields of oncology and antimicrobial research. This article explores the biological activity of neogambogic acid, focusing on its mechanisms of action, therapeutic potential, and relevant case studies.
Overview of Neogambogic Acid
Neogambogic acid is derived from the resin of the Garcinia species, traditionally used in Chinese medicine. It exhibits a range of pharmacological properties, including anti-cancer, anti-inflammatory, and antibacterial activities. Its structure allows it to interact with various biological targets, making it a compound of interest for therapeutic applications.
Neogambogic acid exerts its biological effects through several mechanisms:
- Induction of Apoptosis : NGA has been shown to activate apoptotic pathways in cancer cells. It increases the expression of pro-apoptotic proteins such as Bax and decreases anti-apoptotic proteins like Bcl-2, leading to cell death in various cancer cell lines, including MCF-7 (breast cancer) and HepG2 (liver cancer) .
- Inhibition of Tumor Growth : Studies indicate that NGA inhibits tumor growth by downregulating critical growth factors such as Vascular Endothelial Growth Factor (VEGF) and inhibiting signaling pathways like NF-κB and MAPK . This inhibition leads to reduced proliferation and increased apoptosis in malignant cells.
- Antimicrobial Activity : Recent research highlights NGA's ability to inhibit bacterial growth by targeting undecaprenyl diphosphate synthase (UPPS), an essential enzyme for bacterial cell wall synthesis. It has demonstrated efficacy against antibiotic-resistant strains such as MRSA .
Case Studies
-
Cancer Therapeutics :
- In a study involving human breast cancer cells (MCF-7), NGA induced apoptosis via the activation of caspases and modulation of key signaling pathways. The treatment resulted in significant reductions in cell viability at concentrations as low as 1 µM .
- Another study reported that NGA effectively inhibited the proliferation of pancreatic adenocarcinoma cells, showcasing its potential in treating aggressive cancers .
- Antibacterial Effects :
Data Tables
Biological Activity | Mechanism | Cell Lines Tested | IC50 Values |
---|---|---|---|
Induction of Apoptosis | Upregulation of Bax; Downregulation of Bcl-2 | MCF-7, HepG2 | 1 µM (MCF-7) |
Tumor Growth Inhibition | Inhibition of VEGF; NF-κB signaling | Pancreatic adenocarcinoma | Not specified |
Antibacterial Activity | Inhibition of UPPS | E. faecalis, MRSA | Not specified |
Propriétés
IUPAC Name |
(E)-4-[10,12-dihydroxy-8,21,21-trimethyl-5-(3-methylbut-2-enyl)-8-(4-methylpent-3-enyl)-14,18-dioxo-3,7,20-trioxahexacyclo[15.4.1.02,15.02,19.04,13.06,11]docosa-4(13),5,11,15-tetraen-19-yl]-2-methylbut-2-enoic acid | |
---|---|---|
Source | PubChem | |
URL | https://pubchem.ncbi.nlm.nih.gov | |
Description | Data deposited in or computed by PubChem | |
InChI |
InChI=1S/C38H46O9/c1-19(2)10-9-14-36(8)18-25(39)27-30(41)28-29(40)24-16-22-17-26-35(6,7)47-37(33(22)42,15-13-21(5)34(43)44)38(24,26)46-32(28)23(31(27)45-36)12-11-20(3)4/h10-11,13,16,22,25-26,39,41H,9,12,14-15,17-18H2,1-8H3,(H,43,44)/b21-13+ | |
Source | PubChem | |
URL | https://pubchem.ncbi.nlm.nih.gov | |
Description | Data deposited in or computed by PubChem | |
InChI Key |
BLDWFKHVHHINGR-FYJGNVAPSA-N | |
Source | PubChem | |
URL | https://pubchem.ncbi.nlm.nih.gov | |
Description | Data deposited in or computed by PubChem | |
Canonical SMILES |
CC(=CCCC1(CC(C2=C(C3=C(C(=C2O1)CC=C(C)C)OC45C6CC(C=C4C3=O)C(=O)C5(OC6(C)C)CC=C(C)C(=O)O)O)O)C)C | |
Source | PubChem | |
URL | https://pubchem.ncbi.nlm.nih.gov | |
Description | Data deposited in or computed by PubChem | |
Isomeric SMILES |
CC(=CCCC1(CC(C2=C(C3=C(C(=C2O1)CC=C(C)C)OC45C6CC(C=C4C3=O)C(=O)C5(OC6(C)C)C/C=C(\C)/C(=O)O)O)O)C)C | |
Source | PubChem | |
URL | https://pubchem.ncbi.nlm.nih.gov | |
Description | Data deposited in or computed by PubChem | |
Molecular Formula |
C38H46O9 | |
Source | PubChem | |
URL | https://pubchem.ncbi.nlm.nih.gov | |
Description | Data deposited in or computed by PubChem | |
Molecular Weight |
646.8 g/mol | |
Source | PubChem | |
URL | https://pubchem.ncbi.nlm.nih.gov | |
Description | Data deposited in or computed by PubChem | |
CAS No. |
93772-31-7 | |
Record name | Neo-gambogic acid | |
Source | ChemIDplus | |
URL | https://pubchem.ncbi.nlm.nih.gov/substance/?source=chemidplus&sourceid=0093772317 | |
Description | ChemIDplus is a free, web search system that provides access to the structure and nomenclature authority files used for the identification of chemical substances cited in National Library of Medicine (NLM) databases, including the TOXNET system. | |
Retrosynthesis Analysis
AI-Powered Synthesis Planning: Our tool employs the Template_relevance Pistachio, Template_relevance Bkms_metabolic, Template_relevance Pistachio_ringbreaker, Template_relevance Reaxys, Template_relevance Reaxys_biocatalysis model, leveraging a vast database of chemical reactions to predict feasible synthetic routes.
One-Step Synthesis Focus: Specifically designed for one-step synthesis, it provides concise and direct routes for your target compounds, streamlining the synthesis process.
Accurate Predictions: Utilizing the extensive PISTACHIO, BKMS_METABOLIC, PISTACHIO_RINGBREAKER, REAXYS, REAXYS_BIOCATALYSIS database, our tool offers high-accuracy predictions, reflecting the latest in chemical research and data.
Strategy Settings
Precursor scoring | Relevance Heuristic |
---|---|
Min. plausibility | 0.01 |
Model | Template_relevance |
Template Set | Pistachio/Bkms_metabolic/Pistachio_ringbreaker/Reaxys/Reaxys_biocatalysis |
Top-N result to add to graph | 6 |
Feasible Synthetic Routes
Avertissement et informations sur les produits de recherche in vitro
Veuillez noter que tous les articles et informations sur les produits présentés sur BenchChem sont destinés uniquement à des fins informatives. Les produits disponibles à l'achat sur BenchChem sont spécifiquement conçus pour des études in vitro, qui sont réalisées en dehors des organismes vivants. Les études in vitro, dérivées du terme latin "in verre", impliquent des expériences réalisées dans des environnements de laboratoire contrôlés à l'aide de cellules ou de tissus. Il est important de noter que ces produits ne sont pas classés comme médicaments et n'ont pas reçu l'approbation de la FDA pour la prévention, le traitement ou la guérison de toute condition médicale, affection ou maladie. Nous devons souligner que toute forme d'introduction corporelle de ces produits chez les humains ou les animaux est strictement interdite par la loi. Il est essentiel de respecter ces directives pour assurer la conformité aux normes légales et éthiques en matière de recherche et d'expérimentation.