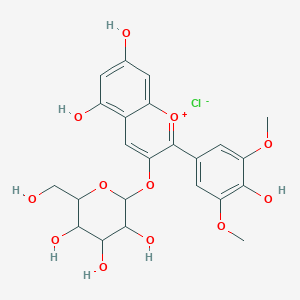
Chlorure d'oenine
Vue d'ensemble
Description
Malvidin 3-O-beta-D-glucoside is an anthocyanin cation consisting of malvidin having a beta-D-glucosyl residue attached at the 3-hydroxy position. It has a role as a metabolite. It is a beta-D-glucoside, an anthocyanin cation and an aromatic ether. It is functionally related to a malvidin. It is a conjugate acid of a malvidin 3-O-beta-D-glucoside betaine.
Oenin is a natural product found in Vaccinium padifolium, Rubus idaeus, and other organisms with data available.
Malvidin 3-O-glucoside is a metabolite found in or produced by Saccharomyces cerevisiae.
Applications De Recherche Scientifique
Propriétés antioxydantes
Le chlorure d'oenine présente une activité antioxydante puissante en raison de sa capacité à piéger les radicaux libres et à protéger les cellules du stress oxydatif. Ses propriétés de piégeage des radicaux contribuent à la santé générale et peuvent aider à prévenir les maladies chroniques .
Effets anti-inflammatoires
La recherche suggère que le this compound possède des propriétés anti-inflammatoires. En modulant les principales voies inflammatoires, il peut aider à atténuer les affections liées à l'inflammation, telles que l'arthrite, les maladies cardiovasculaires et les troubles neurodégénératifs .
Prévention et traitement du cancer
Le this compound est prometteur dans la prévention et le traitement du cancer. Des études ont exploré ses effets sur diverses lignées de cellules cancéreuses, notamment les cellules cancéreuses du côlon (Caco-2) et du foie (HepG2). Il inhibe la prolifération et induit l'apoptose, ce qui en fait un candidat potentiel pour le traitement du cancer .
Neuroprotection
Le malvidin-3-O-glucoside (la forme glycosylée de l'oenine) a été étudié pour ses effets neuroprotecteurs. Il peut atténuer la neurotoxicité induite par le chlorure d'aluminium, ce qui pourrait profiter à la santé du cerveau .
Santé cardiovasculaire
Le this compound contribue à la santé cardiovasculaire en améliorant la fonction endothéliale, en réduisant le stress oxydatif et en améliorant la vasodilatation. Ces effets peuvent aider à prévenir les maladies cardiaques et à promouvoir le bien-être cardiovasculaire général .
Gestion du diabète
Des études ont exploré l'impact du this compound sur le métabolisme du glucose et la sensibilité à l'insuline. Il peut jouer un rôle dans la gestion du diabète en régulant la glycémie et en améliorant la résistance à l'insuline .
En résumé, le this compound, que ce soit sous forme de malvidin-3-O-glucoside ou d'oenine, a un potentiel immense dans des domaines divers, de la protection antioxydante à la thérapie contre le cancer. Ses propriétés multiformes en font un composé précieux pour la recherche scientifique et les applications de santé. 🌟
Mécanisme D'action
Target of Action
Oenin chloride is an anthocyanin, a type of flavonoid that is commonly found in plants . It is the 3-glucoside of malvidin .
Mode of Action
Oenin chloride has been studied for its neuroprotective properties . It has been shown to reduce amyloid β-induced cytotoxicity and diminish reactive oxygen species production in Neuro-2A cells when applied at 50 µM . Additionally, Oenin chloride, at 30 µM, stimulates autophagy in human osteosarcoma U2OS cells .
Biochemical Pathways
Anthocyanins like oenin chloride are known to influence various biochemical pathways, particularly those related to oxidative stress and inflammation .
Result of Action
The molecular and cellular effects of Oenin chloride’s action primarily involve neuroprotection and the stimulation of autophagy . It reduces cytotoxicity induced by amyloid β and diminishes the production of reactive oxygen species . These effects suggest potential therapeutic applications for conditions involving oxidative stress, such as neurodegenerative diseases .
Action Environment
Factors such as ph can influence the stability and color of anthocyanins like oenin chloride .
Analyse Biochimique
Biochemical Properties
Oenin chloride plays a significant role in biochemical reactions, particularly in the stabilization of color in wine. It interacts with various biomolecules, including procyanidin C2, to form stable pigments . The interaction between Oenin chloride and procyanidin C2 results in the formation of a new pigment with a maximal absorption in the red region . Additionally, Oenin chloride is not oxidized in the presence of grape polyphenol oxidase alone but is degraded in the presence of a crude grape PPO extract and caftaric acid, forming anthocyanidin-caftaric acid adducts .
Cellular Effects
Oenin chloride influences various cellular processes. It has been shown to promote resilience against stress by modulating brain synaptic plasticity and peripheral inflammation . This compound affects cell signaling pathways, gene expression, and cellular metabolism, contributing to its neuroprotective effects . In the presence of procyanidin C2, Oenin chloride’s red color appears more stable, indicating its role in cellular interactions and stability .
Molecular Mechanism
At the molecular level, Oenin chloride exerts its effects through binding interactions with biomolecules. It forms stable pigments through self-aggregation of the flavylium cation and copigmentation with the Z-chalcone form . The compound also interacts with procyanidin C2, leading to the formation of new pigments . These interactions contribute to the stabilization of its color and its biochemical properties.
Temporal Effects in Laboratory Settings
In laboratory settings, the effects of Oenin chloride change over time. The compound is relatively stable, but its degradation can occur in the presence of certain enzymes and cofactors . Long-term studies have shown that Oenin chloride can maintain its color stability through interactions with other biomolecules, such as procyanidin C2 . The HPLC chromatogram shows a decrease in the amplitude of the peaks of Oenin chloride and procyanidin C2 over time .
Dosage Effects in Animal Models
The effects of Oenin chloride vary with different dosages in animal models. At lower doses, it has been shown to promote resilience against stress by modulating brain synaptic plasticity and peripheral inflammation . At higher doses, there may be potential toxic or adverse effects, although specific studies on toxicity are limited
Metabolic Pathways
Oenin chloride is involved in various metabolic pathways. It interacts with enzymes such as grape polyphenol oxidase and caftaric acid, leading to the formation of anthocyanidin-caftaric acid adducts . These interactions affect the metabolic flux and metabolite levels, contributing to the compound’s biochemical properties and stability.
Transport and Distribution
Within cells and tissues, Oenin chloride is transported and distributed through interactions with transporters and binding proteins. These interactions influence its localization and accumulation, contributing to its stability and effects on cellular function . The compound’s transport and distribution mechanisms are essential for its role in biochemical reactions and cellular processes.
Subcellular Localization
Oenin chloride’s subcellular localization affects its activity and function. The compound is directed to specific compartments or organelles through targeting signals and post-translational modifications . These localization mechanisms ensure that Oenin chloride exerts its effects in the appropriate cellular contexts, contributing to its biochemical and cellular properties.
Activité Biologique
Oenin, a glycosylated flavonoid primarily found in grape skins and wines, has garnered significant interest due to its diverse biological activities. This article aims to synthesize current research findings on the biological activity of oenin, focusing on its antioxidant, anti-inflammatory, and potential therapeutic effects.
Chemical Structure and Properties
Oenin is a glycoside of the anthocyanin malvin, characterized by its sugar moiety, which enhances its solubility and bioavailability. The molecular formula for oenin is C_22H_23O_11, and its structure includes a glucose unit attached to the anthocyanin backbone.
1. Antioxidant Activity
Oenin exhibits potent antioxidant properties, which are crucial for combating oxidative stress in biological systems. Studies have shown that oenin can effectively scavenge free radicals and inhibit lipid peroxidation, thereby protecting cellular components from oxidative damage.
- Table 1: Antioxidant Activity of Oenin
2. Anti-Inflammatory Effects
Oenin has been reported to exhibit anti-inflammatory properties by modulating various inflammatory pathways. It inhibits the production of pro-inflammatory cytokines such as TNF-α and IL-6.
- Case Study: Inhibition of NF-κB Pathway A study demonstrated that oenin significantly reduced the activation of the NF-κB pathway in macrophages, leading to decreased expression of inflammatory markers .
3. Antimicrobial Properties
Research indicates that oenin possesses antimicrobial activity against various pathogens, including bacteria and fungi. This property is particularly relevant in food preservation and potential therapeutic applications.
-
Table 2: Antimicrobial Activity of Oenin
Pathogen Minimum Inhibitory Concentration (MIC) Escherichia coli 50 µg/mL Staphylococcus aureus 40 µg/mL
The biological activities of oenin can be attributed to several mechanisms:
- Antioxidant Mechanism : Oenin's ability to donate electrons allows it to neutralize free radicals directly.
- Anti-inflammatory Mechanism : By inhibiting NF-κB activation, oenin reduces the transcription of genes involved in inflammation.
- Antimicrobial Mechanism : Oenin disrupts microbial cell membranes and inhibits enzymatic functions critical for microbial survival.
Therapeutic Potential
Given its diverse biological activities, oenin holds promise as a therapeutic agent. Research is ongoing into its potential applications in treating conditions such as:
- Cardiovascular Diseases : Due to its antioxidant properties.
- Chronic Inflammatory Conditions : As an anti-inflammatory agent.
- Infectious Diseases : For its antimicrobial effects.
Propriétés
IUPAC Name |
2-[5,7-dihydroxy-2-(4-hydroxy-3,5-dimethoxyphenyl)chromenylium-3-yl]oxy-6-(hydroxymethyl)oxane-3,4,5-triol;chloride | |
---|---|---|
Details | Computed by Lexichem TK 2.7.0 (PubChem release 2021.05.07) | |
Source | PubChem | |
URL | https://pubchem.ncbi.nlm.nih.gov | |
Description | Data deposited in or computed by PubChem | |
InChI |
InChI=1S/C23H24O12.ClH/c1-31-14-3-9(4-15(32-2)18(14)27)22-16(7-11-12(26)5-10(25)6-13(11)33-22)34-23-21(30)20(29)19(28)17(8-24)35-23;/h3-7,17,19-21,23-24,28-30H,8H2,1-2H3,(H2-,25,26,27);1H | |
Details | Computed by InChI 1.0.6 (PubChem release 2021.05.07) | |
Source | PubChem | |
URL | https://pubchem.ncbi.nlm.nih.gov | |
Description | Data deposited in or computed by PubChem | |
InChI Key |
YDIKCZBMBPOGFT-UHFFFAOYSA-N | |
Details | Computed by InChI 1.0.6 (PubChem release 2021.05.07) | |
Source | PubChem | |
URL | https://pubchem.ncbi.nlm.nih.gov | |
Description | Data deposited in or computed by PubChem | |
Canonical SMILES |
COC1=CC(=CC(=C1O)OC)C2=[O+]C3=CC(=CC(=C3C=C2OC4C(C(C(C(O4)CO)O)O)O)O)O.[Cl-] | |
Details | Computed by OEChem 2.3.0 (PubChem release 2021.05.07) | |
Source | PubChem | |
URL | https://pubchem.ncbi.nlm.nih.gov | |
Description | Data deposited in or computed by PubChem | |
Molecular Formula |
C23H25ClO12 | |
Details | Computed by PubChem 2.1 (PubChem release 2021.05.07) | |
Source | PubChem | |
URL | https://pubchem.ncbi.nlm.nih.gov | |
Description | Data deposited in or computed by PubChem | |
Molecular Weight |
528.9 g/mol | |
Details | Computed by PubChem 2.1 (PubChem release 2021.05.07) | |
Source | PubChem | |
URL | https://pubchem.ncbi.nlm.nih.gov | |
Description | Data deposited in or computed by PubChem | |
CAS No. |
7228-78-6 | |
Record name | Oenin | |
Source | DTP/NCI | |
URL | https://dtp.cancer.gov/dtpstandard/servlet/dwindex?searchtype=NSC&outputformat=html&searchlist=70532 | |
Description | The NCI Development Therapeutics Program (DTP) provides services and resources to the academic and private-sector research communities worldwide to facilitate the discovery and development of new cancer therapeutic agents. | |
Explanation | Unless otherwise indicated, all text within NCI products is free of copyright and may be reused without our permission. Credit the National Cancer Institute as the source. | |
Retrosynthesis Analysis
AI-Powered Synthesis Planning: Our tool employs the Template_relevance Pistachio, Template_relevance Bkms_metabolic, Template_relevance Pistachio_ringbreaker, Template_relevance Reaxys, Template_relevance Reaxys_biocatalysis model, leveraging a vast database of chemical reactions to predict feasible synthetic routes.
One-Step Synthesis Focus: Specifically designed for one-step synthesis, it provides concise and direct routes for your target compounds, streamlining the synthesis process.
Accurate Predictions: Utilizing the extensive PISTACHIO, BKMS_METABOLIC, PISTACHIO_RINGBREAKER, REAXYS, REAXYS_BIOCATALYSIS database, our tool offers high-accuracy predictions, reflecting the latest in chemical research and data.
Strategy Settings
Precursor scoring | Relevance Heuristic |
---|---|
Min. plausibility | 0.01 |
Model | Template_relevance |
Template Set | Pistachio/Bkms_metabolic/Pistachio_ringbreaker/Reaxys/Reaxys_biocatalysis |
Top-N result to add to graph | 6 |
Feasible Synthetic Routes
ANone: Oenin (malvidin 3-O-glucoside) has a molecular formula of C23H25ClO12 and a molecular weight of 532.89 g/mol.
A: Oenin exhibits characteristic absorption maxima in the UV-Vis region, with its major peak around 520 nm, responsible for its red color. [, , ] This absorbance can be influenced by factors like pH, solvent, and the presence of copigments. [, , , , ] Additionally, oenin can be analyzed using techniques like HPLC coupled with UV and fluorescence detectors. []
A: Yes, the color stability of oenin can be significantly improved through copigmentation, a phenomenon where it forms complexes with other molecules like phenolic compounds and flavonoids. [, , , , , ] This interaction often results in a bathochromic shift, enhancing the red color and increasing its resistance to degradation. [, , , ]
A: Research indicates that oenin, along with other anthocyanidins like cyanidin, can inhibit the epidermal growth factor receptor (EGFR), a protein often overexpressed in cancer cells. [] This interaction leads to the inhibition of downstream signaling cascades, potentially contributing to the growth-inhibitory properties of oenin. []
A: Studies using Neuro-2A cells have shown that oenin can protect against neurotoxicity induced by amyloid β-peptide (Aβ), a key player in Alzheimer's disease. [] This protective effect is attributed to oenin's ability to block reactive oxygen species (ROS) formation, preserve calcium homeostasis, and prevent Aβ-mediated gene dysregulation involved in Aβ metabolism and cellular defense. []
A: Oenin, due to its color properties and potential health benefits, finds applications in the food industry as a natural food colorant. [, ] Its antioxidant and anti-inflammatory properties make it a subject of interest for potential use in developing functional foods and nutraceuticals. [, ]
A: Research on the Indian grape cultivar Manjari Medika (MM) revealed a high abundance of oenin, suggesting its potential as a functional food. [] This study highlights the varietal differences in metabolite profiles and emphasizes the importance of understanding these differences for developing novel grape varieties with enhanced nutraceutical properties. []
A: Yes, computational techniques like density functional theory (DFT) have been employed to investigate the interactions between oenin and potential copigments, such as syringic acid and quercetin. [, ] These studies provide insights into the structural features, binding energies, and spectral shifts associated with oenin copigmentation. [, ] Additionally, molecular docking simulations were employed to assess the affinity of oenin and other phytocompounds against specific target proteins of Borrelia recurrentis, the bacterium responsible for louse-borne relapsing fever. []
A: Comparing the activity of oenin (malvidin 3-O-glucoside) with its aglycon, malvidin, reveals the impact of glycosylation on biological activity. Studies show that malvidin exhibits potent inhibitory effects on EGFR tyrosine kinase activity, while oenin shows limited activity. [] This suggests that the presence of the glucoside moiety in oenin may hinder its interaction with the target. []
A: Research suggests that the structure of the copigment significantly affects its ability to complex with oenin. For instance, vinylcatechin dimers exhibit a stronger binding affinity for oenin compared to catechin dimer procyanidin B3, highlighting the importance of the copigment's structure in determining the strength of the interaction. [] Furthermore, the presence of a pyrogallol group in the B ring of flavan-3-ol copigments has been linked to increased copigmentation potential. []
Avertissement et informations sur les produits de recherche in vitro
Veuillez noter que tous les articles et informations sur les produits présentés sur BenchChem sont destinés uniquement à des fins informatives. Les produits disponibles à l'achat sur BenchChem sont spécifiquement conçus pour des études in vitro, qui sont réalisées en dehors des organismes vivants. Les études in vitro, dérivées du terme latin "in verre", impliquent des expériences réalisées dans des environnements de laboratoire contrôlés à l'aide de cellules ou de tissus. Il est important de noter que ces produits ne sont pas classés comme médicaments et n'ont pas reçu l'approbation de la FDA pour la prévention, le traitement ou la guérison de toute condition médicale, affection ou maladie. Nous devons souligner que toute forme d'introduction corporelle de ces produits chez les humains ou les animaux est strictement interdite par la loi. Il est essentiel de respecter ces directives pour assurer la conformité aux normes légales et éthiques en matière de recherche et d'expérimentation.