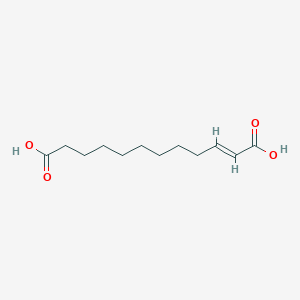
Acide traumatique
Vue d'ensemble
Description
L'acide traumatique, également connu sous le nom d'acide trans-2-dodécènedioïque, est un acide dicarboxylique monoinsaturé qui se trouve naturellement dans les plantes. Il a été isolé pour la première fois à partir de plantes de haricots blessées par les chimistes américains James English Jr. et James Frederick Bonner, ainsi que par le scientifique néerlandais Arie Jan Haagen-Smit, en 1939 . L'this compound est connu pour ses puissantes propriétés cicatrisantes chez les plantes, où il stimule la division cellulaire près d'un site de traumatisme pour former un cal protecteur et guérir les tissus endommagés . Il est également considéré comme une hormone de croissance, en particulier dans les plantes inférieures comme les algues .
Applications De Recherche Scientifique
L'acide traumatique a plusieurs applications en recherche scientifique :
Chimie : Il est utilisé comme intermédiaire dans la synthèse des prostaglandines.
Médecine : Il est un constituant de certains produits pharmaceutiques, comme le gel odontostomatologique Restomyl, en raison de son activité de réépithélialisation muqueuse.
5. Mécanisme d'action
L'this compound exerce ses effets principalement par son rôle d'hormone cicatrisante chez les plantes. Il stimule la division cellulaire près d'un site de traumatisme, conduisant à la formation d'un cal protecteur et à la guérison des tissus endommagés . Les cibles moléculaires et les voies impliquées comprennent l'activation des voies de division et de croissance cellulaires, en particulier en réponse aux blessures . Dans les cellules humaines, l'this compound s'est avéré diminuer la peroxydation des phospholipides membranaires, présenter des propriétés protectrices contre la production d'espèces réactives de l'oxygène et augmenter la biosynthèse des protéines et du collagène .
Mécanisme D'action
Target of Action
Traumatic Acid (TA) is a plant hormone (cytokinin) that belongs to the group of fatty acids derivatives . It primarily targets human skin fibroblasts and stimulates cell division near a trauma site to form a protective callus and heal the damaged tissue . It also acts as a growth hormone, especially in inferior plants (e.g., algae) .
Mode of Action
TA interacts with its targets and causes a decrease in membrane phospholipid peroxidation, exhibiting protective properties against Reactive Oxygen Species (ROS) production . It also increases protein and collagen biosynthesis and its secretion into the culture medium . TA’s activity is similar to unsaturated fatty acids, showing antioxidant and stimulatory effects on collagen biosynthesis .
Biochemical Pathways
TA’s biosynthesis may start either from linoleic acid or linolenic acid, both of which are 18-carbon unsaturated fatty acids precursors . TA influences basic oxidative stress parameters, such as antioxidative enzyme activity, reduced glutathione, thiol group content, and lipid peroxidation .
Result of Action
TA exhibits multiple and complex activity in fibroblast cells in vitro . It reduces oxidative stress and enhances collagen biosynthesis in cultured human skin fibroblasts . It is a potentially powerful agent with applications in the treatment of many skin diseases connected with oxidative stress and collagen biosynthesis disorders .
Action Environment
The action of TA is influenced by the physiological conditions of the environment. For instance, TA’s influence on tested parameters was examined in normal human skin fibroblasts in physiological conditions, without any stress factors . .
Analyse Biochimique
Biochemical Properties
Traumatic acid is biosynthesized in plants by non-enzymatic oxidation of traumatin, another wound hormone . It has been found to enhance the biosynthesis of collagen in cultured human skin fibroblasts . Traumatic acid also exhibits antioxidant properties, reducing membrane phospholipid peroxidation and protecting against reactive oxygen species (ROS) production .
Cellular Effects
Traumatic acid has been shown to have multiple and complex activity in fibroblast cells in vitro . It stimulates cell proliferation and biochemical processes connected with cell division, such as protein, nucleic acid, and photosynthetic dye biosynthesis . In addition, traumatic acid has been found to inhibit MCF-7 breast cancer cells’ viability and enhance apoptosis and oxidative stress .
Molecular Mechanism
It is known that traumatic acid, similar to other unsaturated fatty acids, can activate certain signal transduction pathways for the activation of many metabolic functions in fibroblasts .
Temporal Effects in Laboratory Settings
It has been observed that traumatic acid exhibits a stimulatory effect on various parameters such as antioxidative enzyme activity, reduced glutathione, thiol group content, and lipid peroxidation .
Metabolic Pathways
Traumatic acid is involved in the biosynthesis pathway of traumatin, another wound hormone . It is produced by the non-enzymatic oxidation of traumatin
Transport and Distribution
It is known that traumatic acid is a solid, crystalline, water-insoluble substance under normal conditions .
Subcellular Localization
Given its role as a wound hormone in plants, it is likely to be localized in areas of the cell involved in response to injury and cell division .
Méthodes De Préparation
L'acide traumatique peut être synthétisé par plusieurs méthodes :
Voies de synthèse : Une méthode courante implique la conversion de l'acide undécylènique en demi-aldéhyde de l'acide sébacique, qui est ensuite condensé avec l'acide malonique en présence de pyridine.
Production industrielle : Industriellement, l'this compound est produit par autoxydation de l'acide trans-10-dodécènoïque (trans-10-ODA) en présence d'oxygène et sous agitation rapide. Cette méthode est efficace et donne un produit de haute pureté.
Analyse Des Réactions Chimiques
L'acide traumatique subit diverses réactions chimiques, notamment :
Réduction : Il peut être réduit en ses alcools correspondants dans des conditions spécifiques.
Les réactifs courants utilisés dans ces réactions comprennent l'oxygène pour l'oxydation, les agents réducteurs comme l'hydrure de lithium aluminium pour la réduction, ainsi que les alcools ou les bases pour l'estérification et la formation de sels. Les principaux produits formés à partir de ces réactions comprennent divers esters, sels et formes réduites de l'this compound.
Comparaison Avec Des Composés Similaires
L'acide traumatique est unique en raison de son double rôle d'agent cicatrisant et d'hormone de croissance chez les plantes. Des composés similaires comprennent :
Acide linoléique et acide linolénique : Ce sont des précurseurs dans la biosynthèse de l'this compound et partagent des structures d'acides gras insaturés similaires.
Activité Biologique
Traumatic acid (TA) is a plant-derived compound recognized for its significant biological activities, particularly in the context of wound healing and metabolic regulation. It is classified as a cytokinin and has been isolated from various plant sources, including Phaseolus vulgaris. This article aims to provide a comprehensive overview of the biological activity of traumatic acid, focusing on its antioxidant properties, effects on lipid metabolism, and potential therapeutic applications.
Antioxidant Properties
Traumatic acid exhibits notable antioxidant activity, which has been demonstrated in various studies. It plays a crucial role in reducing oxidative stress by enhancing collagen biosynthesis and stimulating antioxidative enzyme activity. For instance, a study highlighted that TA treatment resulted in decreased lipid peroxidation and increased levels of reduced glutathione in fibroblast cells, indicating its protective effects against reactive oxygen species (ROS) .
Effects on Collagen Biosynthesis
One of the prominent effects of traumatic acid is its stimulatory influence on collagen production. In vitro studies have shown that TA significantly increases collagen content and promotes protein synthesis in human fibroblasts. This activity is comparable to that of unsaturated fatty acids, suggesting that TA could be utilized in therapeutic strategies aimed at enhancing tissue repair and regeneration .
Lipid Metabolism Regulation
Recent research has uncovered traumatic acid's role in lipid metabolism. A study demonstrated that TA treatment led to a significant reduction in lipid accumulation in human adipocytes and prevented obesity induced by a high-fat diet in zebrafish models. The underlying mechanism involves the inhibition of long-chain acyl-CoA synthetase-4 (ACSL4), which is pivotal in fatty acid metabolism . The activation of fatty acid degradation pathways further supports TA's potential as a therapeutic agent against obesity.
Study 1: Antioxidative Effects in Plant Cells
A study investigating the effects of traumatic acid on Wolffia arrhiza revealed that exogenous application of TA significantly enhanced the synthesis of primary metabolites such as proteins, chlorophylls, and carotenoids. Concentrations between M and M were particularly effective, resulting in increased photosynthetic activity and antioxidative enzyme activity, including ascorbate peroxidase and catalase .
Concentration (M) | Protein Synthesis (kDa) | Photosynthesis Intensity | Antioxidative Enzyme Activity |
---|---|---|---|
Control | Control | Control | |
10, 58, 90 | Increased | Increased | |
Increased | Significantly Increased | Significantly Increased |
Study 2: Impact on Lipid Accumulation
In a recent study focused on human adipocytes, traumatic acid was shown to significantly inhibit lipid accumulation. The research utilized transcriptome sequencing to identify pathways activated by TA that are involved in fatty acid metabolism. The findings indicated that TA's interaction with hexokinase 2 (HK2) plays a critical role in mediating these effects .
Toxicological Considerations
While traumatic acid has promising biological activities, it is essential to consider its toxicological profile. Research indicates that high concentrations of TA can exhibit toxic effects when combined with certain herbicides, potentially influencing cancer cell viability . Therefore, understanding the dosage and context of use is crucial for maximizing therapeutic benefits while minimizing risks.
Propriétés
IUPAC Name |
(E)-dodec-2-enedioic acid | |
---|---|---|
Source | PubChem | |
URL | https://pubchem.ncbi.nlm.nih.gov | |
Description | Data deposited in or computed by PubChem | |
InChI |
InChI=1S/C12H20O4/c13-11(14)9-7-5-3-1-2-4-6-8-10-12(15)16/h7,9H,1-6,8,10H2,(H,13,14)(H,15,16)/b9-7+ | |
Source | PubChem | |
URL | https://pubchem.ncbi.nlm.nih.gov | |
Description | Data deposited in or computed by PubChem | |
InChI Key |
MAZWDMBCPDUFDJ-VQHVLOKHSA-N | |
Source | PubChem | |
URL | https://pubchem.ncbi.nlm.nih.gov | |
Description | Data deposited in or computed by PubChem | |
Canonical SMILES |
C(CCCCC(=O)O)CCCC=CC(=O)O | |
Source | PubChem | |
URL | https://pubchem.ncbi.nlm.nih.gov | |
Description | Data deposited in or computed by PubChem | |
Isomeric SMILES |
C(CCCCC(=O)O)CCC/C=C/C(=O)O | |
Source | PubChem | |
URL | https://pubchem.ncbi.nlm.nih.gov | |
Description | Data deposited in or computed by PubChem | |
Molecular Formula |
C12H20O4 | |
Source | PubChem | |
URL | https://pubchem.ncbi.nlm.nih.gov | |
Description | Data deposited in or computed by PubChem | |
DSSTOX Substance ID |
DTXSID00863778 | |
Record name | Traumatic acid | |
Source | EPA DSSTox | |
URL | https://comptox.epa.gov/dashboard/DTXSID00863778 | |
Description | DSSTox provides a high quality public chemistry resource for supporting improved predictive toxicology. | |
Molecular Weight |
228.28 g/mol | |
Source | PubChem | |
URL | https://pubchem.ncbi.nlm.nih.gov | |
Description | Data deposited in or computed by PubChem | |
Physical Description |
Solid | |
Record name | Traumatic acid | |
Source | Human Metabolome Database (HMDB) | |
URL | http://www.hmdb.ca/metabolites/HMDB0000933 | |
Description | The Human Metabolome Database (HMDB) is a freely available electronic database containing detailed information about small molecule metabolites found in the human body. | |
Explanation | HMDB is offered to the public as a freely available resource. Use and re-distribution of the data, in whole or in part, for commercial purposes requires explicit permission of the authors and explicit acknowledgment of the source material (HMDB) and the original publication (see the HMDB citing page). We ask that users who download significant portions of the database cite the HMDB paper in any resulting publications. | |
CAS No. |
6402-36-4 | |
Record name | Traumatic acid | |
Source | CAS Common Chemistry | |
URL | https://commonchemistry.cas.org/detail?cas_rn=6402-36-4 | |
Description | CAS Common Chemistry is an open community resource for accessing chemical information. Nearly 500,000 chemical substances from CAS REGISTRY cover areas of community interest, including common and frequently regulated chemicals, and those relevant to high school and undergraduate chemistry classes. This chemical information, curated by our expert scientists, is provided in alignment with our mission as a division of the American Chemical Society. | |
Explanation | The data from CAS Common Chemistry is provided under a CC-BY-NC 4.0 license, unless otherwise stated. | |
Record name | Traumatic acid | |
Source | ChemIDplus | |
URL | https://pubchem.ncbi.nlm.nih.gov/substance/?source=chemidplus&sourceid=0006402364 | |
Description | ChemIDplus is a free, web search system that provides access to the structure and nomenclature authority files used for the identification of chemical substances cited in National Library of Medicine (NLM) databases, including the TOXNET system. | |
Record name | Traumatic acid | |
Source | EPA DSSTox | |
URL | https://comptox.epa.gov/dashboard/DTXSID00863778 | |
Description | DSSTox provides a high quality public chemistry resource for supporting improved predictive toxicology. | |
Record name | Dodec-2-enedioic acid | |
Source | European Chemicals Agency (ECHA) | |
URL | https://echa.europa.eu/substance-information/-/substanceinfo/100.026.382 | |
Description | The European Chemicals Agency (ECHA) is an agency of the European Union which is the driving force among regulatory authorities in implementing the EU's groundbreaking chemicals legislation for the benefit of human health and the environment as well as for innovation and competitiveness. | |
Explanation | Use of the information, documents and data from the ECHA website is subject to the terms and conditions of this Legal Notice, and subject to other binding limitations provided for under applicable law, the information, documents and data made available on the ECHA website may be reproduced, distributed and/or used, totally or in part, for non-commercial purposes provided that ECHA is acknowledged as the source: "Source: European Chemicals Agency, http://echa.europa.eu/". Such acknowledgement must be included in each copy of the material. ECHA permits and encourages organisations and individuals to create links to the ECHA website under the following cumulative conditions: Links can only be made to webpages that provide a link to the Legal Notice page. | |
Record name | TRAUMATIC ACID | |
Source | FDA Global Substance Registration System (GSRS) | |
URL | https://gsrs.ncats.nih.gov/ginas/app/beta/substances/L7ND24937H | |
Description | The FDA Global Substance Registration System (GSRS) enables the efficient and accurate exchange of information on what substances are in regulated products. Instead of relying on names, which vary across regulatory domains, countries, and regions, the GSRS knowledge base makes it possible for substances to be defined by standardized, scientific descriptions. | |
Explanation | Unless otherwise noted, the contents of the FDA website (www.fda.gov), both text and graphics, are not copyrighted. They are in the public domain and may be republished, reprinted and otherwise used freely by anyone without the need to obtain permission from FDA. Credit to the U.S. Food and Drug Administration as the source is appreciated but not required. | |
Record name | Traumatic acid | |
Source | Human Metabolome Database (HMDB) | |
URL | http://www.hmdb.ca/metabolites/HMDB0000933 | |
Description | The Human Metabolome Database (HMDB) is a freely available electronic database containing detailed information about small molecule metabolites found in the human body. | |
Explanation | HMDB is offered to the public as a freely available resource. Use and re-distribution of the data, in whole or in part, for commercial purposes requires explicit permission of the authors and explicit acknowledgment of the source material (HMDB) and the original publication (see the HMDB citing page). We ask that users who download significant portions of the database cite the HMDB paper in any resulting publications. | |
Melting Point |
165.5 °C | |
Record name | Traumatic acid | |
Source | Human Metabolome Database (HMDB) | |
URL | http://www.hmdb.ca/metabolites/HMDB0000933 | |
Description | The Human Metabolome Database (HMDB) is a freely available electronic database containing detailed information about small molecule metabolites found in the human body. | |
Explanation | HMDB is offered to the public as a freely available resource. Use and re-distribution of the data, in whole or in part, for commercial purposes requires explicit permission of the authors and explicit acknowledgment of the source material (HMDB) and the original publication (see the HMDB citing page). We ask that users who download significant portions of the database cite the HMDB paper in any resulting publications. | |
Retrosynthesis Analysis
AI-Powered Synthesis Planning: Our tool employs the Template_relevance Pistachio, Template_relevance Bkms_metabolic, Template_relevance Pistachio_ringbreaker, Template_relevance Reaxys, Template_relevance Reaxys_biocatalysis model, leveraging a vast database of chemical reactions to predict feasible synthetic routes.
One-Step Synthesis Focus: Specifically designed for one-step synthesis, it provides concise and direct routes for your target compounds, streamlining the synthesis process.
Accurate Predictions: Utilizing the extensive PISTACHIO, BKMS_METABOLIC, PISTACHIO_RINGBREAKER, REAXYS, REAXYS_BIOCATALYSIS database, our tool offers high-accuracy predictions, reflecting the latest in chemical research and data.
Strategy Settings
Precursor scoring | Relevance Heuristic |
---|---|
Min. plausibility | 0.01 |
Model | Template_relevance |
Template Set | Pistachio/Bkms_metabolic/Pistachio_ringbreaker/Reaxys/Reaxys_biocatalysis |
Top-N result to add to graph | 6 |
Feasible Synthetic Routes
Avertissement et informations sur les produits de recherche in vitro
Veuillez noter que tous les articles et informations sur les produits présentés sur BenchChem sont destinés uniquement à des fins informatives. Les produits disponibles à l'achat sur BenchChem sont spécifiquement conçus pour des études in vitro, qui sont réalisées en dehors des organismes vivants. Les études in vitro, dérivées du terme latin "in verre", impliquent des expériences réalisées dans des environnements de laboratoire contrôlés à l'aide de cellules ou de tissus. Il est important de noter que ces produits ne sont pas classés comme médicaments et n'ont pas reçu l'approbation de la FDA pour la prévention, le traitement ou la guérison de toute condition médicale, affection ou maladie. Nous devons souligner que toute forme d'introduction corporelle de ces produits chez les humains ou les animaux est strictement interdite par la loi. Il est essentiel de respecter ces directives pour assurer la conformité aux normes légales et éthiques en matière de recherche et d'expérimentation.