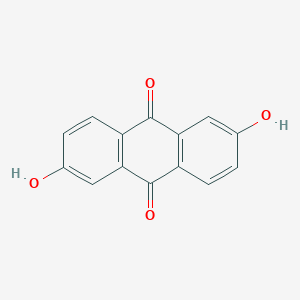
2,6-Dihydroxyanthraquinone
Vue d'ensemble
Description
2,6-Dihydroxyanthraquinone, also known as Anthraflavic acid or Anthraflavin, is an organic compound with the molecular formula C14H8O4 . It is a potent and specific inhibitor of cytochrome P-448 activity . It is a dihydroxyanthraquinone that is anthracene substituted by hydroxy groups at C-3 and C-7 and oxo groups at C-9 and C-10 .
Synthesis Analysis
The synthesis of 2,6-Dihydroxyanthraquinone has been a subject of research. A study published in Nature Communications reported the molecular engineering of dihydroxyanthraquinone-based electrolytes for high-capacity aqueous organic redox flow batteries . Another study discussed the solvation regulation strategy to extend the lifetime of 2,6-dihydroxyanthraquinone (DHAQ) electrolytes .Molecular Structure Analysis
The molecular structure of 2,6-Dihydroxyanthraquinone consists of 14 carbon atoms, 8 hydrogen atoms, and 4 oxygen atoms . The single crystal structure has been investigated by X-ray diffraction and is assigned as monoclinic .Chemical Reactions Analysis
In terms of chemical reactions, a study in Nature Communications initially demonstrated the presence of a hydrogen bond-mediated degradation mechanism of DHAQ molecules during electrochemical reactions . Another study documented the first case of a solvation regulation strategy to extend the lifetime of 2,6-dihydroxyanthraquinone (DHAQ) electrolytes .Physical And Chemical Properties Analysis
2,6-Dihydroxyanthraquinone is a solid substance . It has a molecular weight of 240.21 . It is soluble in DMSO . Its melting point is greater than 320 °C, and it has a boiling point of 342.92°C .Applications De Recherche Scientifique
Flow Batteries
2,6-Dihydroxyanthraquinone (DHAQ) has been studied for its application in flow batteries , particularly in aqueous organic redox flow batteries (AORFBs). Researchers have focused on extending the lifetime of DHAQ electrolytes by incorporating tetramethylammonium cations (TMA+) in the supporting electrolytes. This solvation regulation strategy helps to interfere with the solvation structure of DHAQ anions, thereby deactivating the chemical pathways that lead to decomposition .
Electrochemical Carbon Capture
DHAQ is also being explored for its potential in electrochemical carbon capture . The compound’s ability to undergo redox reactions makes it suitable for capturing and releasing CO2 in aqueous environments. This application is significant for developing sustainable technologies for mitigating climate change impacts .
Stability Enhancement
The molecular structures of anthraquinone derivatives like DHAQ are engineered to maintain long-term stability in aqueous media. This is crucial for their use in various electrochemical applications where stability over time is a key factor .
Energy Storage
In the context of energy storage, DHAQ’s role as a negative electrolyte in AORFBs is vital. Its chemical properties allow for efficient energy storage and release, which is essential for developing more advanced and sustainable battery technologies .
Electrochemical Regeneration
DHAQ’s capacity fade rate has been a subject of study, with research focusing on its dependence on various factors such as hydroxide concentration and state of charge. Understanding these dependencies is important for optimizing the electrochemical regeneration process, where decomposition compounds are oxidized back to active species .
Aqueous Media Applications
The ability of DHAQ to function effectively in aqueous media opens up possibilities for its use in various applications that require water-based solutions. This includes not only batteries but also other technologies that benefit from the compound’s redox properties .
Safety and Hazards
Orientations Futures
The future directions of 2,6-Dihydroxyanthraquinone research are promising. It has been used as a starting material to synthesize tetrahydroxy tetrathiafulvalene (TTF) derivatives, which are used as redox-active building blocks in supramolecular and materials science . A study in Nature Communications reported the molecular engineering of dihydroxyanthraquinone-based electrolytes for high-capacity aqueous organic redox flow batteries . Another study documented the first case of a solvation regulation strategy to extend the lifetime of 2,6-dihydroxyanthraquinone (DHAQ) electrolytes .
Mécanisme D'action
Target of Action
The primary target of 2,6-Dihydroxyanthraquinone, also known as Anthraflavic acid, is cytochrome P-448 . Cytochrome P-448 is an enzyme system closely associated with the activation of many chemical carcinogens .
Mode of Action
Anthraflavic acid is a potent inhibitor of the O-deethylations of ethoxycoumarin and ethoxyresorufin, both catalyzed primarily by cytochromes P-448 . It interferes with the enzyme’s activity, thereby inhibiting the activation of carcinogens.
Biochemical Pathways
It is known to inhibit α-amylase in a cell-free assay This suggests that it may interfere with carbohydrate metabolism
Pharmacokinetics
It is known that the compound’s stability and lifetime can be significantly extended in certain conditions, such as in the presence of tetramethylammonium cations (tma+) in supporting electrolytes .
Result of Action
Anthraflavic acid has been found to have diverse biological activities. It has been shown to inhibit α-amylase and cytochrome P450 activity . It also exhibits cytotoxic effects against various cancer cell lines .
Action Environment
The action, efficacy, and stability of Anthraflavic acid can be influenced by environmental factors. For instance, the presence of tetramethylammonium cations (TMA+) in supporting electrolytes can interfere with the solvation structure of Anthraflavic acid, thereby deactivating the chemical or electrochemical reduction that initiates subsequent side reactions . This strategy is effective when the concentration of Anthraflavic acid is raised to 0.4 M .
Propriétés
IUPAC Name |
2,6-dihydroxyanthracene-9,10-dione | |
---|---|---|
Source | PubChem | |
URL | https://pubchem.ncbi.nlm.nih.gov | |
Description | Data deposited in or computed by PubChem | |
InChI |
InChI=1S/C14H8O4/c15-7-1-3-9-11(5-7)14(18)10-4-2-8(16)6-12(10)13(9)17/h1-6,15-16H | |
Source | PubChem | |
URL | https://pubchem.ncbi.nlm.nih.gov | |
Description | Data deposited in or computed by PubChem | |
InChI Key |
APAJFZPFBHMFQR-UHFFFAOYSA-N | |
Source | PubChem | |
URL | https://pubchem.ncbi.nlm.nih.gov | |
Description | Data deposited in or computed by PubChem | |
Canonical SMILES |
C1=CC2=C(C=C1O)C(=O)C3=C(C2=O)C=C(C=C3)O | |
Source | PubChem | |
URL | https://pubchem.ncbi.nlm.nih.gov | |
Description | Data deposited in or computed by PubChem | |
Molecular Formula |
C14H8O4 | |
Source | PubChem | |
URL | https://pubchem.ncbi.nlm.nih.gov | |
Description | Data deposited in or computed by PubChem | |
DSSTOX Substance ID |
DTXSID6036546 | |
Record name | 2,6-Dihdroxyanthraquinone | |
Source | EPA DSSTox | |
URL | https://comptox.epa.gov/dashboard/DTXSID6036546 | |
Description | DSSTox provides a high quality public chemistry resource for supporting improved predictive toxicology. | |
Molecular Weight |
240.21 g/mol | |
Source | PubChem | |
URL | https://pubchem.ncbi.nlm.nih.gov | |
Description | Data deposited in or computed by PubChem | |
Product Name |
2,6-Dihydroxyanthraquinone | |
CAS RN |
84-60-6 | |
Record name | 2,6-Dihydroxyanthraquinone | |
Source | CAS Common Chemistry | |
URL | https://commonchemistry.cas.org/detail?cas_rn=84-60-6 | |
Description | CAS Common Chemistry is an open community resource for accessing chemical information. Nearly 500,000 chemical substances from CAS REGISTRY cover areas of community interest, including common and frequently regulated chemicals, and those relevant to high school and undergraduate chemistry classes. This chemical information, curated by our expert scientists, is provided in alignment with our mission as a division of the American Chemical Society. | |
Explanation | The data from CAS Common Chemistry is provided under a CC-BY-NC 4.0 license, unless otherwise stated. | |
Record name | 2,6-Dihydroxyanthraquinone | |
Source | ChemIDplus | |
URL | https://pubchem.ncbi.nlm.nih.gov/substance/?source=chemidplus&sourceid=0000084606 | |
Description | ChemIDplus is a free, web search system that provides access to the structure and nomenclature authority files used for the identification of chemical substances cited in National Library of Medicine (NLM) databases, including the TOXNET system. | |
Record name | Anthraflavic acid | |
Source | DTP/NCI | |
URL | https://dtp.cancer.gov/dtpstandard/servlet/dwindex?searchtype=NSC&outputformat=html&searchlist=33531 | |
Description | The NCI Development Therapeutics Program (DTP) provides services and resources to the academic and private-sector research communities worldwide to facilitate the discovery and development of new cancer therapeutic agents. | |
Explanation | Unless otherwise indicated, all text within NCI products is free of copyright and may be reused without our permission. Credit the National Cancer Institute as the source. | |
Record name | 9,10-Anthracenedione, 2,6-dihydroxy- | |
Source | EPA Chemicals under the TSCA | |
URL | https://www.epa.gov/chemicals-under-tsca | |
Description | EPA Chemicals under the Toxic Substances Control Act (TSCA) collection contains information on chemicals and their regulations under TSCA, including non-confidential content from the TSCA Chemical Substance Inventory and Chemical Data Reporting. | |
Record name | 2,6-Dihdroxyanthraquinone | |
Source | EPA DSSTox | |
URL | https://comptox.epa.gov/dashboard/DTXSID6036546 | |
Description | DSSTox provides a high quality public chemistry resource for supporting improved predictive toxicology. | |
Record name | 2,6-dihydroxyanthraquinone | |
Source | European Chemicals Agency (ECHA) | |
URL | https://echa.europa.eu/substance-information/-/substanceinfo/100.001.404 | |
Description | The European Chemicals Agency (ECHA) is an agency of the European Union which is the driving force among regulatory authorities in implementing the EU's groundbreaking chemicals legislation for the benefit of human health and the environment as well as for innovation and competitiveness. | |
Explanation | Use of the information, documents and data from the ECHA website is subject to the terms and conditions of this Legal Notice, and subject to other binding limitations provided for under applicable law, the information, documents and data made available on the ECHA website may be reproduced, distributed and/or used, totally or in part, for non-commercial purposes provided that ECHA is acknowledged as the source: "Source: European Chemicals Agency, http://echa.europa.eu/". Such acknowledgement must be included in each copy of the material. ECHA permits and encourages organisations and individuals to create links to the ECHA website under the following cumulative conditions: Links can only be made to webpages that provide a link to the Legal Notice page. | |
Record name | 2,6-DIHYDROXYANTHRAQUINONE | |
Source | FDA Global Substance Registration System (GSRS) | |
URL | https://gsrs.ncats.nih.gov/ginas/app/beta/substances/W83883330W | |
Description | The FDA Global Substance Registration System (GSRS) enables the efficient and accurate exchange of information on what substances are in regulated products. Instead of relying on names, which vary across regulatory domains, countries, and regions, the GSRS knowledge base makes it possible for substances to be defined by standardized, scientific descriptions. | |
Explanation | Unless otherwise noted, the contents of the FDA website (www.fda.gov), both text and graphics, are not copyrighted. They are in the public domain and may be republished, reprinted and otherwise used freely by anyone without the need to obtain permission from FDA. Credit to the U.S. Food and Drug Administration as the source is appreciated but not required. | |
Synthesis routes and methods
Procedure details
Retrosynthesis Analysis
AI-Powered Synthesis Planning: Our tool employs the Template_relevance Pistachio, Template_relevance Bkms_metabolic, Template_relevance Pistachio_ringbreaker, Template_relevance Reaxys, Template_relevance Reaxys_biocatalysis model, leveraging a vast database of chemical reactions to predict feasible synthetic routes.
One-Step Synthesis Focus: Specifically designed for one-step synthesis, it provides concise and direct routes for your target compounds, streamlining the synthesis process.
Accurate Predictions: Utilizing the extensive PISTACHIO, BKMS_METABOLIC, PISTACHIO_RINGBREAKER, REAXYS, REAXYS_BIOCATALYSIS database, our tool offers high-accuracy predictions, reflecting the latest in chemical research and data.
Strategy Settings
Precursor scoring | Relevance Heuristic |
---|---|
Min. plausibility | 0.01 |
Model | Template_relevance |
Template Set | Pistachio/Bkms_metabolic/Pistachio_ringbreaker/Reaxys/Reaxys_biocatalysis |
Top-N result to add to graph | 6 |
Feasible Synthetic Routes
Q & A
ANone: Anthraflavic acid exhibits its effects through various mechanisms depending on the target.
- Inhibition of α-amylase: Molecular docking studies have shown that anthraflavic acid exhibits a considerable binding affinity to α-amylase, suggesting its potential as an inhibitor. [, ] This inhibition can be beneficial in managing Type 2 diabetes mellitus.
- Cytotoxic effects on breast cancer cells: Anthraflavic acid has demonstrated significant cytotoxic effects against various human breast carcinoma cell lines (MCF-7, CAMA-1, SK-BR-3, MDA-MB-231, AU565, and Hs 281.T) in a time- and concentration-dependent manner. [, ] This anti-cancer effect is suggested to be linked to its antioxidant properties. []
- Inhibition of epidermal xenobiotic metabolism: Anthraflavic acid, along with other plant phenols, has been found to inhibit epidermal cytochrome P450-dependent monooxygenases, specifically aryl hydrocarbon hydroxylase (AHH) activity. [] This inhibition can potentially reduce the carcinogenic effects of polycyclic aromatic hydrocarbons (PAHs) in the skin.
A:
- Spectroscopic data: Anthraflavic acid has been characterized using various spectroscopic techniques, including UV-Vis spectroscopy, Fourier transform infrared (FTIR) spectroscopy, and nuclear magnetic resonance (NMR) spectroscopy. [, , ]
ANone: Anthraflavic acid has been successfully incorporated into various materials and applications:
- Thin films: Anthraflavic acid has been used as a dopant in thin films of silicon phthalocyanines for potential use in luminescent devices. [] It has also been used in the synthesis of charge-transfer donor-acceptor systems based on Curtis-type complexes for conductivity measurements in thin-film form. []
- Solid-state devices: Anthraflavic acid-doped titanyl/vanadyl phthalocyanine has been used to fabricate solid-state devices on recycled Tetrapak and graphite electrodes. []
- Redox flow batteries: Anthraflavic acid has shown promise as an anolyte material in alkaline redox flow batteries, particularly when paired with ferrocyanide as the catholyte. [, , ]
ANone:
- Molecular docking: This method has been employed to study the interaction of anthraflavic acid with α-amylase, revealing its potential as an enzyme inhibitor. [, ]
- Density Functional Theory (DFT): DFT calculations have been used to investigate the ground state hydrogen conformations and vibrational analysis of anthraflavic acid isomers. []
- Molecular modeling: This technique has been utilized to understand the increased thermal stabilities of DNA G-quadruplexes with anthraquinone insertions, including those derived from anthraflavic acid. []
A: Studies have shown that the position of hydroxyl groups on the anthraquinone ring significantly influences the biological activity of anthraflavic acid and its isomers. [, ] For instance, while anthraflavic acid (2,6-dihydroxyanthraquinone) exhibits inhibitory effects on PAH metabolism, other dihydroxyanthraquinone isomers like 1,4-dihydroxyanthraquinone have different activities. [, ] Additionally, the presence and type of substituents on the anthracene molecule impact its binding affinity to human serum albumin. []
A: Research suggests that anthraflavic acid can degrade in alkaline solutions used in redox flow batteries, highlighting the need for stabilization strategies. [] Furthermore, exploring various formulation approaches, such as encapsulation techniques, might be beneficial to improve solubility, bioavailability, and stability for different applications.
Avertissement et informations sur les produits de recherche in vitro
Veuillez noter que tous les articles et informations sur les produits présentés sur BenchChem sont destinés uniquement à des fins informatives. Les produits disponibles à l'achat sur BenchChem sont spécifiquement conçus pour des études in vitro, qui sont réalisées en dehors des organismes vivants. Les études in vitro, dérivées du terme latin "in verre", impliquent des expériences réalisées dans des environnements de laboratoire contrôlés à l'aide de cellules ou de tissus. Il est important de noter que ces produits ne sont pas classés comme médicaments et n'ont pas reçu l'approbation de la FDA pour la prévention, le traitement ou la guérison de toute condition médicale, affection ou maladie. Nous devons souligner que toute forme d'introduction corporelle de ces produits chez les humains ou les animaux est strictement interdite par la loi. Il est essentiel de respecter ces directives pour assurer la conformité aux normes légales et éthiques en matière de recherche et d'expérimentation.