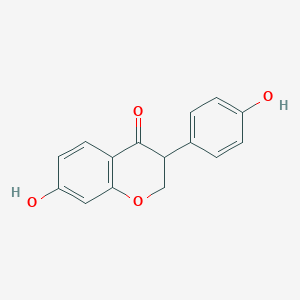
Dihydrodaidzéine
Vue d'ensemble
Description
La dihydrodaidzéine est un composé isoflavonique principalement dérivé des principaux constituants des isoflavones de soja, en particulier de la daidzéine, par hydrogénation lors du métabolisme . Elle est connue pour son activité biologique significative et est considérée comme l'une des formes les plus actives des isoflavones de soja .
Applications De Recherche Scientifique
Dihydrodaidzein has a wide range of scientific research applications due to its significant biological activity:
Chemistry: Used in the study of isoflavone metabolism and biotransformation.
Biology: Plays a role in the metabolism of dietary isoflavonoids by gut bacteria.
Medicine: Exhibits antioxidant, cardiovascular disease prevention, and osteoporosis prevention activities.
Industry: Potential applications in the development of functional foods and nutraceuticals.
Mécanisme D'action
Target of Action
Dihydrodaidzein is an active metabolite of daidzein . It primarily targets estrogen receptors and has a vasodilatory action on rat isolated aortic rings . It stimulates the estrogen receptor-dependent growth of breast cancer MCF-7 cells at micromolar concentrations .
Mode of Action
Dihydrodaidzein interacts with its targets, primarily estrogen receptors, leading to changes in cellular function. It stimulates the estrogen receptor-dependent growth of breast cancer MCF-7 cells . The exact molecular interactions between dihydrodaidzein and its targets are still under investigation.
Biochemical Pathways
Dihydrodaidzein is primarily derived from the principal constituents of soy isoflavones—daidzein, through hydrogenation in metabolism . It is produced by the metabolism of daidzein in colonic bacteria and may be further metabolized to various bioactive compounds, including equol . Daidzin is first deglycosylated to large amounts of daidzein by almost all the isolated bacteria and subsequently to minor amounts of dihydrodaidzein through methoxylation and hydroxylation by few bacteria .
Pharmacokinetics
Dihydrodaidzein suffers from low water solubility, instability, and low oral bioavailability . It is mainly derived from biosynthesis, and it can also be produced by chemical synthesis and enzyme catalyzation . Dihydrodaidzein is detected in the plasma 4 hours after the intravenous injection . It can penetrate the blood-brain barrier .
Result of Action
The molecular and cellular effects of dihydrodaidzein’s action are primarily related to its interaction with estrogen receptors. It stimulates the estrogen receptor-dependent growth of breast cancer MCF-7 cells . It also has vasodilatory action on rat isolated aortic rings .
Action Environment
The action, efficacy, and stability of dihydrodaidzein are influenced by environmental factors such as the presence of intestinal microorganisms. These microorganisms play a crucial role in the metabolic transformation of daidzein to dihydrodaidzein . The conversion of daidzein to dihydrodaidzein can be influenced by the specific strains of bacteria present in the gut .
Analyse Biochimique
Biochemical Properties
Dihydrodaidzein interacts with various enzymes, proteins, and other biomolecules. It is mainly derived from biosynthesis, and can also be produced by chemical synthesis and enzyme catalyzation . Intestinal bacteria play a pivotal role in the biotransformation of daidzein, the precursor of Dihydrodaidzein, and the enrichment and substantial synthesis of DHD depend on the selection of highly efficient bacterial strains from the multitude participating in daidzein conversion .
Cellular Effects
Dihydrodaidzein has been found to exhibit higher and broader biological activity compared to its metabolic precursor daidzein, such as improved osteoporosis activity . It has vasodilatory action on rat isolated aortic rings and stimulates the estrogen receptor-dependent growth of breast cancer MCF-7 cells at micromolar concentrations .
Molecular Mechanism
The molecular mechanism of Dihydrodaidzein involves its interaction with biomolecules and changes in gene expression. For instance, it has been found that Dihydrodaidzein can reversely convert into daidzein even without cofactors under aerobic conditions . This suggests that Dihydrodaidzein may have a role in regulating the levels of daidzein in the body.
Temporal Effects in Laboratory Settings
It has been observed that Dihydrodaidzein can be detected in the plasma 4 hours after intravenous injection
Metabolic Pathways
Dihydrodaidzein is involved in several metabolic pathways. It is primarily derived from the hydrogenation of daidzein in metabolism . The conversion of daidzein to Dihydrodaidzein is primarily achieved through the secretion of a series of enzymes, with different enzymes required at different stages .
Transport and Distribution
It has been found that Dihydrodaidzein and its derivatives could be detected in the plasma and in organs except the heart . This suggests that Dihydrodaidzein may be widely distributed within the body.
Méthodes De Préparation
Voies de synthèse et conditions de réaction : La dihydrodaidzéine peut être synthétisée par synthèse chimique et catalyse enzymatique. La voie de biosynthèse implique la conversion de la daidzéine en this compound par les bactéries intestinales, qui jouent un rôle crucial dans le processus de biotransformation . La sélection de souches bactériennes hautement efficaces est cruciale pour l'enrichissement et la synthèse substantielle de la this compound .
Méthodes de production industrielle : Dans les milieux industriels, la this compound peut être produite en utilisant une nouvelle bactérie du rumen bovin tolérante à l'oxygène capable de bioconvertir de manière anaérobie les isoflavones daidzéine et génistéine en this compound et dihydrogénistéine, respectivement . Le taux de bioconversion peut être amélioré en ajoutant de l'acide ascorbique au milieu de culture .
Analyse Des Réactions Chimiques
Types de réactions : La dihydrodaidzéine subit diverses réactions chimiques, notamment la réduction et la racémisation. Par exemple, elle peut être convertie en tétrahydrodaidzéine par une réaction de réduction diastéréosélective . De plus, la racémase de this compound peut convertir à la fois les énantiomères ®- et (S)- de la this compound en racémate .
Réactifs et conditions courants :
Réduction : La réductase de this compound (DHDR) est utilisée pour la réduction de la this compound en tétrahydrodaidzéine.
Racémisation : La racémase de this compound est essentielle pour le processus de racémisation.
Principaux produits :
Tétrahydrodaidzéine : Formée par la réduction de la this compound.
Équol : Produit à partir de la this compound par une série de réactions enzymatiques.
4. Applications de la recherche scientifique
La this compound a une large gamme d'applications de recherche scientifique en raison de son activité biologique significative :
Chimie : Utilisée dans l'étude du métabolisme et de la biotransformation des isoflavones.
Biologie : Joue un rôle dans le métabolisme des isoflavonoïdes alimentaires par les bactéries intestinales.
Industrie : Applications potentielles dans le développement d'aliments fonctionnels et de nutraceutiques.
5. Mécanisme d'action
La this compound exerce ses effets par son interaction avec le microbiote intestinal. Elle est métabolisée par les bactéries intestinales pour produire des métabolites tels que la tétrahydrodaidzéine et l'équol, qui présentent des activités biologiques améliorées . Le mécanisme implique la conversion de la this compound en ces métabolites par une série de réactions enzymatiques, notamment la réduction et la racémisation .
Composés similaires :
Daidzéine : Le précurseur de la this compound, également une isoflavone à activité biologique significative.
Génistéine : Une autre isoflavone dérivée du soja, similaire en structure et en fonction à la this compound.
Équol : Un métabolite de la this compound avec une activité estrogénique puissante.
Unicité : La this compound est unique en raison de son activité biologique plus élevée et plus large comparée à son précurseur métabolique, la daidzéine . Elle présente une activité anti-ostéoporose améliorée et d'autres avantages pour la santé, ce qui en fait un composé d'un intérêt majeur dans la recherche scientifique .
Comparaison Avec Des Composés Similaires
Daidzein: The precursor of dihydrodaidzein, also an isoflavone with significant biological activity.
Genistein: Another isoflavone derived from soy, similar in structure and function to dihydrodaidzein.
Equol: A metabolite of dihydrodaidzein with potent estrogenic activity.
Uniqueness: Dihydrodaidzein is unique due to its higher and broader biological activity compared to its metabolic precursor daidzein . It exhibits improved osteoporosis activity and other health benefits, making it a compound of significant interest in scientific research .
Activité Biologique
Dihydrodaidzein is a metabolite of daidzein, a soy isoflavone, and has garnered attention for its potential biological activities, particularly concerning human health. This article synthesizes current research findings on the biological activity of dihydrodaidzein, including its metabolic pathways, effects on gut microbiota, and implications for human health.
Metabolism and Conversion
Dihydrodaidzein is primarily produced from daidzein through the action of gut microbiota. The conversion of daidzein to dihydrodaidzein is facilitated by specific bacteria in the intestinal tract. Notably, a study identified a strain of Lactococcus that possesses a novel enzyme, dihydrodaidzein racemase (l-DDRC), which plays a crucial role in the biosynthesis of equol from dihydrodaidzein. This enzyme allows for the conversion of both enantiomers of dihydrodaidzein into racemic mixtures, which are further metabolized into equol, a compound with significant biological activity .
Biological Activities
Dihydrodaidzein exhibits several biological activities that contribute to its potential health benefits:
- Antioxidant Activity : Dihydrodaidzein has been shown to possess antioxidant properties, which help in neutralizing free radicals and reducing oxidative stress in cells. This activity is crucial for preventing cellular damage and may play a role in reducing the risk of chronic diseases .
- Anticancer Properties : Research indicates that dihydrodaidzein may exhibit antiproliferative effects against various tumor cells. It has been linked to inhibiting cancer cell growth through mechanisms such as apoptosis induction and cell cycle arrest .
- Estrogenic Effects : As a phytoestrogen, dihydrodaidzein can mimic estrogen in the body, potentially influencing hormonal balance and providing benefits in conditions such as menopausal symptoms and osteoporosis .
Interaction with Gut Microbiota
The metabolism of dihydrodaidzein is significantly influenced by gut microbiota. Studies have shown that specific bacterial strains can enhance the conversion of dietary isoflavones into bioactive metabolites like equol. For instance, Clostridium sp. strain Julong 732 was identified as capable of converting dihydrodaidzein into equol under anaerobic conditions . This conversion not only enhances the bioavailability of these compounds but also contributes to the overall health-promoting effects associated with dietary isoflavones.
Case Studies and Research Findings
Several studies have investigated the effects and mechanisms of action of dihydrodaidzein:
- Study on Antioxidant Activity : A study demonstrated that dihydrodaidzein effectively scavenged free radicals in vitro, suggesting its potential as a natural antioxidant agent .
- Anticancer Mechanism : In vitro experiments showed that dihydrodaidzein inhibited the proliferation of breast cancer cells by inducing apoptosis and modulating cell cycle progression .
- Microbial Conversion Studies : Research involving fecal microbiota transplantation revealed that adding isoflavone-metabolizing bacteria could significantly enhance the metabolism of dietary isoflavones, leading to increased levels of bioactive metabolites like equol and potentially improving health outcomes .
Summary Table: Biological Activities of Dihydrodaidzein
Propriétés
IUPAC Name |
7-hydroxy-3-(4-hydroxyphenyl)-2,3-dihydrochromen-4-one | |
---|---|---|
Source | PubChem | |
URL | https://pubchem.ncbi.nlm.nih.gov | |
Description | Data deposited in or computed by PubChem | |
InChI |
InChI=1S/C15H12O4/c16-10-3-1-9(2-4-10)13-8-19-14-7-11(17)5-6-12(14)15(13)18/h1-7,13,16-17H,8H2 | |
Source | PubChem | |
URL | https://pubchem.ncbi.nlm.nih.gov | |
Description | Data deposited in or computed by PubChem | |
InChI Key |
JHYXBPPMXZIHKG-UHFFFAOYSA-N | |
Source | PubChem | |
URL | https://pubchem.ncbi.nlm.nih.gov | |
Description | Data deposited in or computed by PubChem | |
Canonical SMILES |
C1C(C(=O)C2=C(O1)C=C(C=C2)O)C3=CC=C(C=C3)O | |
Source | PubChem | |
URL | https://pubchem.ncbi.nlm.nih.gov | |
Description | Data deposited in or computed by PubChem | |
Molecular Formula |
C15H12O4 | |
Source | PubChem | |
URL | https://pubchem.ncbi.nlm.nih.gov | |
Description | Data deposited in or computed by PubChem | |
DSSTOX Substance ID |
DTXSID70912308 | |
Record name | Dihydrodaidzein | |
Source | EPA DSSTox | |
URL | https://comptox.epa.gov/dashboard/DTXSID70912308 | |
Description | DSSTox provides a high quality public chemistry resource for supporting improved predictive toxicology. | |
Molecular Weight |
256.25 g/mol | |
Source | PubChem | |
URL | https://pubchem.ncbi.nlm.nih.gov | |
Description | Data deposited in or computed by PubChem | |
Physical Description |
Solid | |
Record name | Dihydrodaidzein | |
Source | Human Metabolome Database (HMDB) | |
URL | http://www.hmdb.ca/metabolites/HMDB0005760 | |
Description | The Human Metabolome Database (HMDB) is a freely available electronic database containing detailed information about small molecule metabolites found in the human body. | |
Explanation | HMDB is offered to the public as a freely available resource. Use and re-distribution of the data, in whole or in part, for commercial purposes requires explicit permission of the authors and explicit acknowledgment of the source material (HMDB) and the original publication (see the HMDB citing page). We ask that users who download significant portions of the database cite the HMDB paper in any resulting publications. | |
CAS No. |
17238-05-0 | |
Record name | (±)-Dihydrodaidzein | |
Source | CAS Common Chemistry | |
URL | https://commonchemistry.cas.org/detail?cas_rn=17238-05-0 | |
Description | CAS Common Chemistry is an open community resource for accessing chemical information. Nearly 500,000 chemical substances from CAS REGISTRY cover areas of community interest, including common and frequently regulated chemicals, and those relevant to high school and undergraduate chemistry classes. This chemical information, curated by our expert scientists, is provided in alignment with our mission as a division of the American Chemical Society. | |
Explanation | The data from CAS Common Chemistry is provided under a CC-BY-NC 4.0 license, unless otherwise stated. | |
Record name | Isoflavanone, 4',7-dihydroxy- | |
Source | ChemIDplus | |
URL | https://pubchem.ncbi.nlm.nih.gov/substance/?source=chemidplus&sourceid=0017238050 | |
Description | ChemIDplus is a free, web search system that provides access to the structure and nomenclature authority files used for the identification of chemical substances cited in National Library of Medicine (NLM) databases, including the TOXNET system. | |
Record name | Dihydrodaidzein | |
Source | EPA DSSTox | |
URL | https://comptox.epa.gov/dashboard/DTXSID70912308 | |
Description | DSSTox provides a high quality public chemistry resource for supporting improved predictive toxicology. | |
Record name | Dihydrodaidzein | |
Source | Human Metabolome Database (HMDB) | |
URL | http://www.hmdb.ca/metabolites/HMDB0005760 | |
Description | The Human Metabolome Database (HMDB) is a freely available electronic database containing detailed information about small molecule metabolites found in the human body. | |
Explanation | HMDB is offered to the public as a freely available resource. Use and re-distribution of the data, in whole or in part, for commercial purposes requires explicit permission of the authors and explicit acknowledgment of the source material (HMDB) and the original publication (see the HMDB citing page). We ask that users who download significant portions of the database cite the HMDB paper in any resulting publications. | |
Melting Point |
250 °C | |
Record name | Dihydrodaidzein | |
Source | Human Metabolome Database (HMDB) | |
URL | http://www.hmdb.ca/metabolites/HMDB0005760 | |
Description | The Human Metabolome Database (HMDB) is a freely available electronic database containing detailed information about small molecule metabolites found in the human body. | |
Explanation | HMDB is offered to the public as a freely available resource. Use and re-distribution of the data, in whole or in part, for commercial purposes requires explicit permission of the authors and explicit acknowledgment of the source material (HMDB) and the original publication (see the HMDB citing page). We ask that users who download significant portions of the database cite the HMDB paper in any resulting publications. | |
Synthesis routes and methods I
Procedure details
Synthesis routes and methods II
Procedure details
Retrosynthesis Analysis
AI-Powered Synthesis Planning: Our tool employs the Template_relevance Pistachio, Template_relevance Bkms_metabolic, Template_relevance Pistachio_ringbreaker, Template_relevance Reaxys, Template_relevance Reaxys_biocatalysis model, leveraging a vast database of chemical reactions to predict feasible synthetic routes.
One-Step Synthesis Focus: Specifically designed for one-step synthesis, it provides concise and direct routes for your target compounds, streamlining the synthesis process.
Accurate Predictions: Utilizing the extensive PISTACHIO, BKMS_METABOLIC, PISTACHIO_RINGBREAKER, REAXYS, REAXYS_BIOCATALYSIS database, our tool offers high-accuracy predictions, reflecting the latest in chemical research and data.
Strategy Settings
Precursor scoring | Relevance Heuristic |
---|---|
Min. plausibility | 0.01 |
Model | Template_relevance |
Template Set | Pistachio/Bkms_metabolic/Pistachio_ringbreaker/Reaxys/Reaxys_biocatalysis |
Top-N result to add to graph | 6 |
Feasible Synthetic Routes
Avertissement et informations sur les produits de recherche in vitro
Veuillez noter que tous les articles et informations sur les produits présentés sur BenchChem sont destinés uniquement à des fins informatives. Les produits disponibles à l'achat sur BenchChem sont spécifiquement conçus pour des études in vitro, qui sont réalisées en dehors des organismes vivants. Les études in vitro, dérivées du terme latin "in verre", impliquent des expériences réalisées dans des environnements de laboratoire contrôlés à l'aide de cellules ou de tissus. Il est important de noter que ces produits ne sont pas classés comme médicaments et n'ont pas reçu l'approbation de la FDA pour la prévention, le traitement ou la guérison de toute condition médicale, affection ou maladie. Nous devons souligner que toute forme d'introduction corporelle de ces produits chez les humains ou les animaux est strictement interdite par la loi. Il est essentiel de respecter ces directives pour assurer la conformité aux normes légales et éthiques en matière de recherche et d'expérimentation.