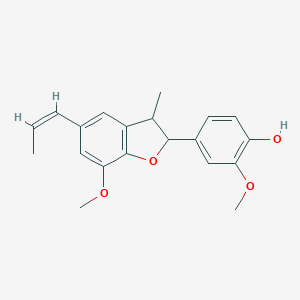
Dehydrodiisoeugenol
Vue d'ensemble
Description
Dehydrodiisoeugenol (DHIE) is a neolignan found in more than 17 plant species, including herbs, fruit, and root . It was first isolated from Myristica fragrans bark in 1973 .
Synthesis Analysis
DHIE has been obtained using various methodologies, including classical chemistry synthesis using metal catalysts and biocatalytic synthesis . Biocatalysis is an excellent option to obtain DHIE as it does not use metal catalysts and operates under mild and environmentally friendly reaction conditions . The yield of DHIE by biocatalysis employed different sources for enzymes as catalysts and produced a yield range of 20 to 98% .Molecular Structure Analysis
The molecular formula of DHIE is C20H22O4 . It was determined before it was isolated from a natural source .Chemical Reactions Analysis
Various extraction methods have been used globally for the extraction of eugenol and other nutraceutics from plants . The most extensively employed approaches include solvent extraction, hydro-distillation, microwave-assisted extraction, supercritical carbon dioxide extraction, and ultrasound-based extraction .Physical And Chemical Properties Analysis
The molecular weight of DHIE is 326.4 g/mol . More detailed physical and chemical properties are not available in the retrieved papers.Applications De Recherche Scientifique
Source and Synthesis
DHIE is a neolignan found in more than 17 plant species, including herbs, fruit, and root . It was first isolated from Myristica fragrans bark in 1973 . Since then, many methodologies have been used for the synthesis of DHIE, including classical chemistry synthesis using metal catalysts and biocatalytic synthesis . Some of the biocatalysts employed include horseradish peroxidase, peroxidase from Cocos nucifera, laccase, culture cells of plants, and microorganisms .
Anti-Inflammatory Properties
DHIE has been found to have anti-inflammatory properties . This makes it potentially useful in the treatment of conditions characterized by inflammation.
Anti-Oxidant Properties
DHIE also exhibits anti-oxidant properties . Antioxidants are substances that can prevent or slow damage to cells caused by free radicals, unstable molecules that the body produces as a reaction to environmental and other pressures.
Anti-Cancerogenic Properties
Research has indicated that DHIE has anti-cancerogenic properties . This suggests that it could potentially be used in the development of treatments for various types of cancer.
Anti-Microbial Properties
DHIE has been found to have anti-microbial properties . This means it could potentially be used in the treatment of various bacterial and fungal infections.
Potential Therapeutic Agent
Despite the wide range of biological activities exhibited by DHIE, evidence in vivo and in human beings is still lacking to support the usefulness potential of DHIE as a therapeutic agent . Further research is needed to fully understand its potential applications in medicine.
Orientations Futures
Mécanisme D'action
Dehydrodiisoeugenol (DHIE) is a neolignan found in more than 17 plant species, including herbs, fruit, and root . It was first isolated from Myristica fragrans bark in 1973 . Since then, it has been recognized for its wide range of biological activities .
Target of Action
DHIE has been shown to have anti-inflammatory, anti-oxidant, anti-cancerogenic, and anti-microbial properties . It inhibits the expression of COX-2 in RAW264.7 murine macrophages stimulated with LPS . It also inhibits LPS-stimulated phosphorylation-dependent proteolysis of inhibitor κB-α and transcriptional activity of NF-κB in the cells .
Mode of Action
The mode of action of DHIE involves the inhibition of key inflammatory and oxidative stress pathways. By inhibiting the expression of COX-2 and the activity of NF-κB, DHIE can reduce inflammation and oxidative stress .
Biochemical Pathways
DHIE is a neolignan, a type of secondary metabolite found in plants and animals . These compounds play an essential role in plants’ defenses against microorganisms . Neolignans are dimers of phenylpropane originating from the shikimic acid pathway , and deamination of phenylalanine leads to caffeic acid .
Result of Action
Treatment with DHIE has been shown to arrest the cell cycle of colorectal cancer cells at the G1/S phase, leading to significant inhibition in cell growth . Moreover, DHIE induces strong cellular autophagy .
Action Environment
The action of DHIE can be influenced by various environmental factors. For instance, the use of coconut water as a catalyst yielded 60% of the enantiomer (−)-DHIE . This suggests that the environment in which DHIE is synthesized or administered can affect its efficacy and stability.
Propriétés
IUPAC Name |
2-methoxy-4-[7-methoxy-3-methyl-5-[(E)-prop-1-enyl]-2,3-dihydro-1-benzofuran-2-yl]phenol | |
---|---|---|
Source | PubChem | |
URL | https://pubchem.ncbi.nlm.nih.gov | |
Description | Data deposited in or computed by PubChem | |
InChI |
InChI=1S/C20H22O4/c1-5-6-13-9-15-12(2)19(24-20(15)18(10-13)23-4)14-7-8-16(21)17(11-14)22-3/h5-12,19,21H,1-4H3/b6-5+ | |
Source | PubChem | |
URL | https://pubchem.ncbi.nlm.nih.gov | |
Description | Data deposited in or computed by PubChem | |
InChI Key |
ITDOFWOJEDZPCF-AATRIKPKSA-N | |
Source | PubChem | |
URL | https://pubchem.ncbi.nlm.nih.gov | |
Description | Data deposited in or computed by PubChem | |
Canonical SMILES |
CC=CC1=CC2=C(C(=C1)OC)OC(C2C)C3=CC(=C(C=C3)O)OC | |
Source | PubChem | |
URL | https://pubchem.ncbi.nlm.nih.gov | |
Description | Data deposited in or computed by PubChem | |
Isomeric SMILES |
C/C=C/C1=CC2=C(C(=C1)OC)OC(C2C)C3=CC(=C(C=C3)O)OC | |
Source | PubChem | |
URL | https://pubchem.ncbi.nlm.nih.gov | |
Description | Data deposited in or computed by PubChem | |
Molecular Formula |
C20H22O4 | |
Source | PubChem | |
URL | https://pubchem.ncbi.nlm.nih.gov | |
Description | Data deposited in or computed by PubChem | |
Molecular Weight |
326.4 g/mol | |
Source | PubChem | |
URL | https://pubchem.ncbi.nlm.nih.gov | |
Description | Data deposited in or computed by PubChem | |
Product Name |
Dehydrodiisoeugenol | |
CAS RN |
2680-81-1 | |
Record name | Dehydrodiisoeugenol | |
Source | ChemIDplus | |
URL | https://pubchem.ncbi.nlm.nih.gov/substance/?source=chemidplus&sourceid=0002680811 | |
Description | ChemIDplus is a free, web search system that provides access to the structure and nomenclature authority files used for the identification of chemical substances cited in National Library of Medicine (NLM) databases, including the TOXNET system. | |
Retrosynthesis Analysis
AI-Powered Synthesis Planning: Our tool employs the Template_relevance Pistachio, Template_relevance Bkms_metabolic, Template_relevance Pistachio_ringbreaker, Template_relevance Reaxys, Template_relevance Reaxys_biocatalysis model, leveraging a vast database of chemical reactions to predict feasible synthetic routes.
One-Step Synthesis Focus: Specifically designed for one-step synthesis, it provides concise and direct routes for your target compounds, streamlining the synthesis process.
Accurate Predictions: Utilizing the extensive PISTACHIO, BKMS_METABOLIC, PISTACHIO_RINGBREAKER, REAXYS, REAXYS_BIOCATALYSIS database, our tool offers high-accuracy predictions, reflecting the latest in chemical research and data.
Strategy Settings
Precursor scoring | Relevance Heuristic |
---|---|
Min. plausibility | 0.01 |
Model | Template_relevance |
Template Set | Pistachio/Bkms_metabolic/Pistachio_ringbreaker/Reaxys/Reaxys_biocatalysis |
Top-N result to add to graph | 6 |
Feasible Synthetic Routes
Avertissement et informations sur les produits de recherche in vitro
Veuillez noter que tous les articles et informations sur les produits présentés sur BenchChem sont destinés uniquement à des fins informatives. Les produits disponibles à l'achat sur BenchChem sont spécifiquement conçus pour des études in vitro, qui sont réalisées en dehors des organismes vivants. Les études in vitro, dérivées du terme latin "in verre", impliquent des expériences réalisées dans des environnements de laboratoire contrôlés à l'aide de cellules ou de tissus. Il est important de noter que ces produits ne sont pas classés comme médicaments et n'ont pas reçu l'approbation de la FDA pour la prévention, le traitement ou la guérison de toute condition médicale, affection ou maladie. Nous devons souligner que toute forme d'introduction corporelle de ces produits chez les humains ou les animaux est strictement interdite par la loi. Il est essentiel de respecter ces directives pour assurer la conformité aux normes légales et éthiques en matière de recherche et d'expérimentation.