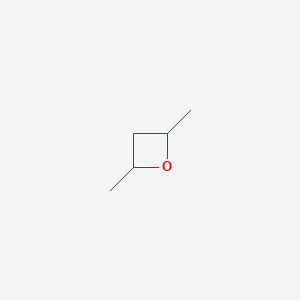
2,4-Dimethyloxetane
Vue d'ensemble
Description
Synthesis Analysis
The synthesis of 2,4-Dimethyloxetane can occur through epoxidation or from reactions of alkoxy radicals . Photolytically induced isomerization of organic peroxy radicals into QOOH may provide an additional source of cyclic ethers in the troposphere .Molecular Structure Analysis
2,4-Dimethyloxetane has two diastereomers, syn-DMO and anti-DMO . The stereochemistry of the peroxy radical determines which QOOH isomerization steps are possible .Chemical Reactions Analysis
The pressure- and temperature-dependent kinetics of seven cyclic ether peroxy radicals, which stem from 2,4-Dimethyloxetane via H-abstraction and O2 addition, were determined . Diastereomeric cyclic ether peroxy radicals show significantly different reactivities .Physical And Chemical Properties Analysis
The physical and chemical properties of 2,4-Dimethyloxetane are largely determined by its molecular structure and the nature of its reactions. For instance, its reactivity can vary significantly depending on the stereochemistry of the peroxy radical .Applications De Recherche Scientifique
Combustion Chemistry and Kinetics :
- 2,4-Dimethyloxetane is significant in the low-temperature combustion of n-pentane, serving as a cyclic ether intermediate formed from hydroperoxyalkyl radicals. Research on its peroxy radicals has revealed stereochemistry-dependent reactivities and complex reaction pathways, which are critical for understanding combustion processes and developing detailed mechanisms for cyclic ether intermediates (Doner, Zádor, & Rotavera, 2022).
Polymer Science :
- Studies on the copolymerization of oxetane and 2,4-Dimethyloxetane have provided insights into reactivity parameters and microstructures of the resulting copolymers, contributing to advanced material science (Bucquoye & Goethals, 1978).
- Polymorphism in poly(3,3-dimethyloxetane) has been explored to understand the effects of crystallization temperatures and kinetic factors on polymer forms, which is crucial for material engineering and applications (Pérez et al., 1985).
Chemical Kinetics and Reaction Mechanisms :
- The synthesis and characterization of star-shaped poly(3,3-dimethyloxetane) involved studying the cationic ring-opening polymerization process. Insights from this research contribute to understanding polymer formation mechanisms (Guo, Zou, & Pan, 2001).
- Detailed studies on the unimolecular reactions of 2,4-dimethyloxetanyl radicals have been conducted to understand their role in combustion and atmospheric chemistry, particularly in the oxidation of cyclic ethers (Doner, Zádor, & Rotavera, 2023).
Spectroscopy and Molecular Structure :
- Research on intermolecular hydrogen bonding in molecules with large amplitude motions, like the rotational spectrum of the 3,3-dimethyloxetane complex, has provided insights into molecular interactions and structural dynamics (Sánchez et al., 2005).
Mécanisme D'action
Propriétés
IUPAC Name |
2,4-dimethyloxetane | |
---|---|---|
Source | PubChem | |
URL | https://pubchem.ncbi.nlm.nih.gov | |
Description | Data deposited in or computed by PubChem | |
InChI |
InChI=1S/C5H10O/c1-4-3-5(2)6-4/h4-5H,3H2,1-2H3 | |
Source | PubChem | |
URL | https://pubchem.ncbi.nlm.nih.gov | |
Description | Data deposited in or computed by PubChem | |
InChI Key |
KPPWZEMUMPFHEX-UHFFFAOYSA-N | |
Source | PubChem | |
URL | https://pubchem.ncbi.nlm.nih.gov | |
Description | Data deposited in or computed by PubChem | |
Canonical SMILES |
CC1CC(O1)C | |
Source | PubChem | |
URL | https://pubchem.ncbi.nlm.nih.gov | |
Description | Data deposited in or computed by PubChem | |
Molecular Formula |
C5H10O | |
Source | PubChem | |
URL | https://pubchem.ncbi.nlm.nih.gov | |
Description | Data deposited in or computed by PubChem | |
DSSTOX Substance ID |
DTXSID40336698 | |
Record name | Oxetane, 2,4-dimethyl- | |
Source | EPA DSSTox | |
URL | https://comptox.epa.gov/dashboard/DTXSID40336698 | |
Description | DSSTox provides a high quality public chemistry resource for supporting improved predictive toxicology. | |
Molecular Weight |
86.13 g/mol | |
Source | PubChem | |
URL | https://pubchem.ncbi.nlm.nih.gov | |
Description | Data deposited in or computed by PubChem | |
Product Name |
2,4-Dimethyloxetane | |
CAS RN |
14988-66-0 | |
Record name | Oxetane, 2,4-dimethyl- | |
Source | EPA DSSTox | |
URL | https://comptox.epa.gov/dashboard/DTXSID40336698 | |
Description | DSSTox provides a high quality public chemistry resource for supporting improved predictive toxicology. | |
Retrosynthesis Analysis
AI-Powered Synthesis Planning: Our tool employs the Template_relevance Pistachio, Template_relevance Bkms_metabolic, Template_relevance Pistachio_ringbreaker, Template_relevance Reaxys, Template_relevance Reaxys_biocatalysis model, leveraging a vast database of chemical reactions to predict feasible synthetic routes.
One-Step Synthesis Focus: Specifically designed for one-step synthesis, it provides concise and direct routes for your target compounds, streamlining the synthesis process.
Accurate Predictions: Utilizing the extensive PISTACHIO, BKMS_METABOLIC, PISTACHIO_RINGBREAKER, REAXYS, REAXYS_BIOCATALYSIS database, our tool offers high-accuracy predictions, reflecting the latest in chemical research and data.
Strategy Settings
Precursor scoring | Relevance Heuristic |
---|---|
Min. plausibility | 0.01 |
Model | Template_relevance |
Template Set | Pistachio/Bkms_metabolic/Pistachio_ringbreaker/Reaxys/Reaxys_biocatalysis |
Top-N result to add to graph | 6 |
Feasible Synthetic Routes
Q & A
Q1: How is 2,4-dimethyloxetane formed during fuel combustion?
A1: 2,4-Dimethyloxetane is a cyclic ether that forms during the low-temperature combustion of certain fuels, particularly n-pentane. [, ] Its formation is linked to the unimolecular reactions of hydroperoxyalkyl radicals (QOOH), specifically those derived from n-pentane. These QOOH radicals undergo an isomerization step involving a six-membered transition state, ultimately leading to the formation of 2,4-dimethyloxetane. []
Q2: Why is understanding the fate of 2,4-dimethyloxetane important in combustion modeling?
A2: 2,4-Dimethyloxetane doesn't just sit inertly in the combustion environment. It can undergo further reactions that influence the overall reaction mechanism and product distribution. [] Critically, it can react via two main pathways:
- Unimolecular Ring-Opening: The oxetane ring can break apart, leading to various products depending on the specific radical formed and the reaction conditions. [] These ring-opening reactions have rates comparable to reactions with oxygen, making them significant competitors. []
- Reaction with Oxygen: 2,4-Dimethyloxetane can react with molecular oxygen to form cyclic ether-peroxy adducts, further complicating the reaction network. []
Q3: What makes the study of 2,4-dimethyloxetane radicals complex?
A3: Several factors contribute to the complexity:
- Stereoisomerism: 2,4-Dimethyloxetane can exist as different stereoisomers, and the stereochemistry of the molecule significantly affects the reactivity of its derived radicals. For instance, diastereomeric cyclic ether peroxy radicals (formed from 2,4-dimethyloxetane) exhibit vastly different reactivity profiles. []
- Multiple Reaction Channels: The potential energy surfaces of these radicals are complex, with multiple accessible reaction pathways leading to a diverse array of products. [, ] This includes conventional QOOH decomposition pathways (like HO2 elimination) and ring-opening reactions, which can lead to unique unsaturated peroxy radicals. []
- Well-Skipping Reactions: At atmospheric pressure, the rate of chemical reactions for many of these radicals is comparable to the rate of collisional stabilization. This leads to "well-skipping" reactions, where the energized radicals react before being fully thermalized, further complicating kinetic analysis. []
Avertissement et informations sur les produits de recherche in vitro
Veuillez noter que tous les articles et informations sur les produits présentés sur BenchChem sont destinés uniquement à des fins informatives. Les produits disponibles à l'achat sur BenchChem sont spécifiquement conçus pour des études in vitro, qui sont réalisées en dehors des organismes vivants. Les études in vitro, dérivées du terme latin "in verre", impliquent des expériences réalisées dans des environnements de laboratoire contrôlés à l'aide de cellules ou de tissus. Il est important de noter que ces produits ne sont pas classés comme médicaments et n'ont pas reçu l'approbation de la FDA pour la prévention, le traitement ou la guérison de toute condition médicale, affection ou maladie. Nous devons souligner que toute forme d'introduction corporelle de ces produits chez les humains ou les animaux est strictement interdite par la loi. Il est essentiel de respecter ces directives pour assurer la conformité aux normes légales et éthiques en matière de recherche et d'expérimentation.