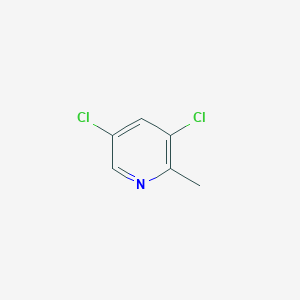
3,5-Dichloro-2-méthylpyridine
Vue d'ensemble
Description
3,5-Dichloro-2-methylpyridine is a chlorinated pyridine derivative with the molecular formula C6H5Cl2N. This compound is characterized by the presence of two chlorine atoms at the 3rd and 5th positions and a methyl group at the 2nd position of the pyridine ring. It is a valuable intermediate in organic synthesis and finds applications in various fields, including pharmaceuticals, agrochemicals, and material science.
Applications De Recherche Scientifique
3,5-Dichloro-2-methylpyridine is utilized in several scientific research applications:
Chemistry: It serves as a building block for the synthesis of more complex organic molecules.
Biology: It is used in the development of bioactive compounds and as a precursor for pharmaceuticals.
Medicine: The compound is investigated for its potential therapeutic properties and as an intermediate in drug synthesis.
Industry: It is employed in the production of agrochemicals, dyes, and specialty chemicals.
Mécanisme D'action
Target of Action
3,5-Dichloro-2-methylpyridine is a highly substituted pyridine derivative. It’s known that pyridine derivatives can interact with various biological targets, including enzymes and receptors, depending on their specific substitutions .
Mode of Action
It’s known that pyridine derivatives can undergo various chemical reactions, including organometallic reactions . For instance, they can react with organomagnesiums and organolithiums to form substituted and functionalized structures . This suggests that 3,5-Dichloro-2-methylpyridine might interact with its targets through similar chemical reactions, leading to changes in the targets’ structure and function.
Biochemical Pathways
It’s known that pyridine derivatives can be involved in various biochemical processes, such as the suzuki–miyaura cross-coupling reaction . This reaction is a widely-used method for forming carbon-carbon bonds, suggesting that 3,5-Dichloro-2-methylpyridine might affect biochemical pathways involving carbon-carbon bond formation .
Result of Action
Given its potential involvement in carbon-carbon bond formation , it might influence the synthesis of various biomolecules, potentially leading to changes in cellular functions and processes.
Méthodes De Préparation
Synthetic Routes and Reaction Conditions: The synthesis of 3,5-Dichloro-2-methylpyridine typically involves the chlorination of 2-methylpyridine. One common method is the direct chlorination using chlorine gas in the presence of a catalyst such as iron(III) chloride. The reaction is carried out under controlled conditions to ensure selective chlorination at the desired positions.
Industrial Production Methods: In an industrial setting, the production of 3,5-Dichloro-2-methylpyridine can be achieved through a multi-step process involving the initial chlorination of 2-methylpyridine followed by purification steps to isolate the desired product. The use of continuous flow reactors and advanced separation techniques can enhance the efficiency and yield of the production process.
Analyse Des Réactions Chimiques
Types of Reactions: 3,5-Dichloro-2-methylpyridine undergoes various chemical reactions, including:
Nucleophilic Substitution: The chlorine atoms can be replaced by nucleophiles such as amines, thiols, or alkoxides.
Oxidation: The methyl group can be oxidized to form corresponding carboxylic acids or aldehydes.
Reduction: The compound can be reduced to form 3,5-dichloro-2-methylpiperidine.
Common Reagents and Conditions:
Nucleophilic Substitution: Reagents like sodium methoxide or potassium tert-butoxide in polar aprotic solvents.
Oxidation: Reagents such as potassium permanganate or chromium trioxide.
Reduction: Catalysts like palladium on carbon (Pd/C) in the presence of hydrogen gas.
Major Products:
- Substituted pyridines with various functional groups.
- Oxidized derivatives such as carboxylic acids or aldehydes.
- Reduced derivatives like piperidines.
Comparaison Avec Des Composés Similaires
- 3,5-Dichloro-2,4,6-trifluoropyridine
- 3-Chloro-2,4,5,6-tetrafluoropyridine
- 2,3-Dichloro-5-methylpyridine
Comparison: 3,5-Dichloro-2-methylpyridine is unique due to its specific substitution pattern, which imparts distinct chemical and physical properties Compared to other chlorinated pyridines, it offers a balance of reactivity and stability, making it suitable for various synthetic applications
Activité Biologique
3,5-Dichloro-2-methylpyridine (DCMP) is a chlorinated pyridine derivative that has garnered attention due to its diverse biological activities and potential applications in medicinal chemistry. This article explores its biological activity, mechanisms of action, and relevant research findings.
Chemical Structure and Properties
3,5-Dichloro-2-methylpyridine is characterized by the presence of two chlorine atoms at the 3 and 5 positions of the pyridine ring, along with a methyl group at the 2 position. Its molecular formula is , and it has a molecular weight of 162.02 g/mol. The compound's structure influences its reactivity and biological interactions.
The biological activity of DCMP is primarily attributed to its ability to interact with various biological targets, including enzymes and receptors. The chlorine substituents and the pyridine nitrogen play crucial roles in modulating these interactions:
- Enzyme Inhibition : DCMP has been shown to inhibit specific enzymes by binding to their active sites or allosteric sites, thereby altering their function. This property is significant in drug development, particularly for targeting diseases where enzyme activity is dysregulated.
- Receptor Binding : The compound exhibits affinity for certain receptors, which can lead to physiological responses such as modulation of neurotransmitter systems or hormonal pathways.
Antimicrobial Activity
Research indicates that DCMP possesses antimicrobial properties. It has been evaluated against various bacterial strains, showing effectiveness in inhibiting growth. Studies suggest that the compound disrupts bacterial cell wall synthesis or interferes with metabolic pathways essential for bacterial survival.
Anticancer Properties
DCMP has also been investigated for its anticancer potential. In vitro studies demonstrate that it can induce apoptosis in cancer cell lines through mechanisms such as oxidative stress and disruption of mitochondrial function. For example, one study reported that DCMP exhibited cytotoxic effects on breast cancer cells, leading to reduced cell viability and increased apoptosis markers .
Case Studies and Research Findings
Several studies have highlighted the biological activity of 3,5-Dichloro-2-methylpyridine:
-
Antimicrobial Efficacy :
- A study evaluated DCMP against Staphylococcus aureus and Escherichia coli, revealing minimum inhibitory concentrations (MICs) of 32 µg/mL for both strains, indicating moderate antibacterial activity.
- Anticancer Activity :
-
Mechanistic Insights :
- Research involving molecular docking simulations indicated that DCMP binds effectively to the active site of certain kinases involved in cancer progression, providing a rationale for its observed anticancer effects.
Comparative Analysis with Similar Compounds
To understand the uniqueness of DCMP's biological activity, it is useful to compare it with similar compounds:
Compound Name | Structure Characteristics | Biological Activity |
---|---|---|
2,3-Dichloro-5-methylpyridine | One chlorine atom less | Moderate antimicrobial activity |
2,4-Dichloro-5-methylpyridine | Different chlorine positioning | Lower anticancer activity compared to DCMP |
3,5-Dichloro-2,4,6-trifluoropyridine | Additional fluorine substituents | Enhanced selectivity but reduced overall potency |
This table illustrates how structural variations influence the biological activities of these compounds.
Propriétés
IUPAC Name |
3,5-dichloro-2-methylpyridine | |
---|---|---|
Source | PubChem | |
URL | https://pubchem.ncbi.nlm.nih.gov | |
Description | Data deposited in or computed by PubChem | |
InChI |
InChI=1S/C6H5Cl2N/c1-4-6(8)2-5(7)3-9-4/h2-3H,1H3 | |
Source | PubChem | |
URL | https://pubchem.ncbi.nlm.nih.gov | |
Description | Data deposited in or computed by PubChem | |
InChI Key |
FPTUCCXFFWYEOU-UHFFFAOYSA-N | |
Source | PubChem | |
URL | https://pubchem.ncbi.nlm.nih.gov | |
Description | Data deposited in or computed by PubChem | |
Canonical SMILES |
CC1=C(C=C(C=N1)Cl)Cl | |
Source | PubChem | |
URL | https://pubchem.ncbi.nlm.nih.gov | |
Description | Data deposited in or computed by PubChem | |
Molecular Formula |
C6H5Cl2N | |
Source | PubChem | |
URL | https://pubchem.ncbi.nlm.nih.gov | |
Description | Data deposited in or computed by PubChem | |
DSSTOX Substance ID |
DTXSID80627931 | |
Record name | 3,5-Dichloro-2-methylpyridine | |
Source | EPA DSSTox | |
URL | https://comptox.epa.gov/dashboard/DTXSID80627931 | |
Description | DSSTox provides a high quality public chemistry resource for supporting improved predictive toxicology. | |
Molecular Weight |
162.01 g/mol | |
Source | PubChem | |
URL | https://pubchem.ncbi.nlm.nih.gov | |
Description | Data deposited in or computed by PubChem | |
CAS No. |
100868-45-9 | |
Record name | 3,5-Dichloro-2-methylpyridine | |
Source | EPA DSSTox | |
URL | https://comptox.epa.gov/dashboard/DTXSID80627931 | |
Description | DSSTox provides a high quality public chemistry resource for supporting improved predictive toxicology. | |
Synthesis routes and methods I
Procedure details
Synthesis routes and methods II
Procedure details
Synthesis routes and methods III
Procedure details
Avertissement et informations sur les produits de recherche in vitro
Veuillez noter que tous les articles et informations sur les produits présentés sur BenchChem sont destinés uniquement à des fins informatives. Les produits disponibles à l'achat sur BenchChem sont spécifiquement conçus pour des études in vitro, qui sont réalisées en dehors des organismes vivants. Les études in vitro, dérivées du terme latin "in verre", impliquent des expériences réalisées dans des environnements de laboratoire contrôlés à l'aide de cellules ou de tissus. Il est important de noter que ces produits ne sont pas classés comme médicaments et n'ont pas reçu l'approbation de la FDA pour la prévention, le traitement ou la guérison de toute condition médicale, affection ou maladie. Nous devons souligner que toute forme d'introduction corporelle de ces produits chez les humains ou les animaux est strictement interdite par la loi. Il est essentiel de respecter ces directives pour assurer la conformité aux normes légales et éthiques en matière de recherche et d'expérimentation.