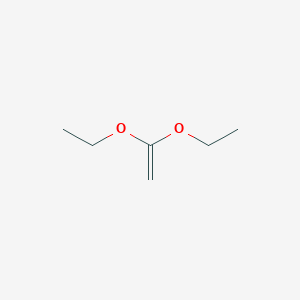
1,1-Diéthoxyéthène
Vue d'ensemble
Description
1,1-Diethoxyethene, also known as Acetaldehyde diethyl acetal or simply Acetal, is a compound that contains an acetal functional group . It is a major flavoring component of distilled beverages, especially malt whisky and sherry .
Synthesis Analysis
The synthesis of 1,1-Diethoxyethene has been studied extensively. One method involves the reaction of isopropanol decomposition, which is used to classify different catalysts used in the synthesis of 1,1-Diethoxyethene . The production of 1,1-Diethoxyethene is clearly correlated with the acidity of the solids .Molecular Structure Analysis
The molecular formula of 1,1-Diethoxyethene is C6H12O2 . It has an average mass of 116.158 Da and a monoisotopic mass of 116.083733 Da .Chemical Reactions Analysis
The direct conversion of ethanol to 1,1-Diethoxyethene (DEE) through one-pot dehydrogenation-acetalization has attracted broad interest from both academia and industry . This process involves the oxidative dehydrogenation of alcohol to acetaldehyde, which requires high temperature to activate oxygen to realize the C−H cleavage, while the acetalization of acetaldehyde with ethanol is an exothermic reversible reaction favorable at low temperature .Physical and Chemical Properties Analysis
1,1-Diethoxyethene has a density of 0.9±0.1 g/cm3, a boiling point of 105.6±13.0 °C at 760 mmHg, and a vapor pressure of 34.1±0.2 mmHg at 25°C . It has an enthalpy of vaporization of 33.0±3.0 kJ/mol, a flash point of 7.0±19.4 °C, and an index of refraction of 1.402 .Applications De Recherche Scientifique
1,1-Diéthoxyéthène: Applications en recherche scientifique: Le this compound, également connu sous le nom de DEE, est un composé chimique doté de diverses applications en recherche scientifique. Voici quelques-unes de ses applications uniques :
Recherche en catalyse
Le DEE est utilisé dans des études impliquant la catalyse bifonctionnelle. Les chercheurs ont rapporté sa synthèse à partir d’éthanol à l’aide d’une voie de déshydrogénation oxydative-acétalisation monotope facilitée par des catalyseurs Bi/BiCeOx .
Applications pharmaceutiques
Il a des applications multidisciplinaires, y compris dans l’industrie pharmaceutique, où il est utilisé comme produit dérivé à valeur ajoutée de l’oxydation sélective de l’éthanol .
Études de synthèse
Le DEE est synthétisé à partir d’éthanol à l’aide de catalyseurs contenant du cuivre. Ce procédé est important dans la recherche sur les méthodes de synthèse en une étape .
Analyse des données thermodynamiques
Les données thermodynamiques du composé, telles que sa chaleur de combustion et de formation, sont essentielles dans divers domaines de la recherche scientifique, y compris les études énergétiques et la science des matériaux .
Mécanisme D'action
- DEE primarily interacts with biological systems through its acetal functional group. It is a major flavoring component in distilled beverages, especially malt whisky and sherry .
Target of Action
Mode of Action
Safety and Hazards
1,1-Diethoxyethene is a highly flammable liquid and vapor. It causes skin irritation and serious eye irritation. It is harmful if inhaled and may damage fertility . It is advised to avoid breathing vapors, mist, or gas, ensure adequate ventilation, remove all sources of ignition, and evacuate personnel to safe areas .
Orientations Futures
The future directions of 1,1-Diethoxyethene research could involve further exploration of its synthesis methods, particularly in relation to the use of different catalysts and the optimization of reaction conditions. Additionally, the potential applications of 1,1-Diethoxyethene in various industries, such as the beverage industry, could be further investigated .
Propriétés
IUPAC Name |
1,1-diethoxyethene | |
---|---|---|
Source | PubChem | |
URL | https://pubchem.ncbi.nlm.nih.gov | |
Description | Data deposited in or computed by PubChem | |
InChI |
InChI=1S/C6H12O2/c1-4-7-6(3)8-5-2/h3-5H2,1-2H3 | |
Source | PubChem | |
URL | https://pubchem.ncbi.nlm.nih.gov | |
Description | Data deposited in or computed by PubChem | |
InChI Key |
VTGIVYVOVVQLRL-UHFFFAOYSA-N | |
Source | PubChem | |
URL | https://pubchem.ncbi.nlm.nih.gov | |
Description | Data deposited in or computed by PubChem | |
Canonical SMILES |
CCOC(=C)OCC | |
Source | PubChem | |
URL | https://pubchem.ncbi.nlm.nih.gov | |
Description | Data deposited in or computed by PubChem | |
Molecular Formula |
C6H12O2 | |
Source | PubChem | |
URL | https://pubchem.ncbi.nlm.nih.gov | |
Description | Data deposited in or computed by PubChem | |
DSSTOX Substance ID |
DTXSID50181292 | |
Record name | Ethene, 1,1-diethoxy- | |
Source | EPA DSSTox | |
URL | https://comptox.epa.gov/dashboard/DTXSID50181292 | |
Description | DSSTox provides a high quality public chemistry resource for supporting improved predictive toxicology. | |
Molecular Weight |
116.16 g/mol | |
Source | PubChem | |
URL | https://pubchem.ncbi.nlm.nih.gov | |
Description | Data deposited in or computed by PubChem | |
CAS No. |
2678-54-8 | |
Record name | Ethene, 1,1-diethoxy- | |
Source | ChemIDplus | |
URL | https://pubchem.ncbi.nlm.nih.gov/substance/?source=chemidplus&sourceid=0002678548 | |
Description | ChemIDplus is a free, web search system that provides access to the structure and nomenclature authority files used for the identification of chemical substances cited in National Library of Medicine (NLM) databases, including the TOXNET system. | |
Record name | Ethene, 1,1-diethoxy- | |
Source | EPA DSSTox | |
URL | https://comptox.epa.gov/dashboard/DTXSID50181292 | |
Description | DSSTox provides a high quality public chemistry resource for supporting improved predictive toxicology. | |
Record name | Ketene diethyl acetal | |
Source | European Chemicals Agency (ECHA) | |
URL | https://echa.europa.eu/information-on-chemicals | |
Description | The European Chemicals Agency (ECHA) is an agency of the European Union which is the driving force among regulatory authorities in implementing the EU's groundbreaking chemicals legislation for the benefit of human health and the environment as well as for innovation and competitiveness. | |
Explanation | Use of the information, documents and data from the ECHA website is subject to the terms and conditions of this Legal Notice, and subject to other binding limitations provided for under applicable law, the information, documents and data made available on the ECHA website may be reproduced, distributed and/or used, totally or in part, for non-commercial purposes provided that ECHA is acknowledged as the source: "Source: European Chemicals Agency, http://echa.europa.eu/". Such acknowledgement must be included in each copy of the material. ECHA permits and encourages organisations and individuals to create links to the ECHA website under the following cumulative conditions: Links can only be made to webpages that provide a link to the Legal Notice page. | |
Retrosynthesis Analysis
AI-Powered Synthesis Planning: Our tool employs the Template_relevance Pistachio, Template_relevance Bkms_metabolic, Template_relevance Pistachio_ringbreaker, Template_relevance Reaxys, Template_relevance Reaxys_biocatalysis model, leveraging a vast database of chemical reactions to predict feasible synthetic routes.
One-Step Synthesis Focus: Specifically designed for one-step synthesis, it provides concise and direct routes for your target compounds, streamlining the synthesis process.
Accurate Predictions: Utilizing the extensive PISTACHIO, BKMS_METABOLIC, PISTACHIO_RINGBREAKER, REAXYS, REAXYS_BIOCATALYSIS database, our tool offers high-accuracy predictions, reflecting the latest in chemical research and data.
Strategy Settings
Precursor scoring | Relevance Heuristic |
---|---|
Min. plausibility | 0.01 |
Model | Template_relevance |
Template Set | Pistachio/Bkms_metabolic/Pistachio_ringbreaker/Reaxys/Reaxys_biocatalysis |
Top-N result to add to graph | 6 |
Feasible Synthetic Routes
Q1: How does 1,1-diethoxyethene interact with quinones, and what are the downstream effects?
A1: 1,1-Diethoxyethene adds to quinones through both 1:1 and 1:2 pathways. [, ] This reaction is particularly useful in synthesizing natural products like acetylemodin and deoxyerythrolaccin, pigments found in insects. [, , ] The 1:2 addition pathway involves the formation of isolable intermediate adducts. []
Q2: What is the structure of 1,1-diethoxyethene, and what is known about its spectroscopic data?
A2: 1,1-Diethoxyethene's molecular formula is C₆H₁₂O₂, and its molecular weight is 116.16 g/mol. [] While the provided research doesn't detail specific spectroscopic data, it's typically characterized using NMR spectroscopy and mass spectrometry.
Q3: How does the structure of 1,1-diethoxyethene influence its reactivity in cycloaddition reactions?
A3: 1,1-Diethoxyethene participates in both [2+2] and [4+2] cycloaddition reactions, showcasing its versatility. [, , , ] Interestingly, the regioselectivity of its reaction with tropone can be influenced by Lewis acid catalysts. For example, B(C6F5)3 favors [4+2] cycloaddition, while BPh3 promotes [8+2] cycloaddition. [] This difference arises from the Lewis acid's impact on the nucleophilicity of the carbonyl oxygen in tropone. []
Q4: Has computational chemistry been used to study 1,1-diethoxyethene reactions?
A4: Yes, density functional theory (DFT) calculations at the ωB97X-D level have been employed to investigate the mechanism of 1,1-diethoxyethene cycloaddition with tropone in the presence of Lewis acid catalysts. [] These calculations provided insights into the reaction pathway and the influence of different Lewis acids on regioselectivity.
Q5: Can 1,1-diethoxyethene be used in photochemical reactions?
A5: Absolutely! 1,1-Diethoxyethene participates in photochemical [2+2] cycloadditions. [, ] One study explored its use in synthesizing DL-apiose derivatives through photochemical reactions with 1,3-dihydroxypropan-2-one derivatives. [] It also undergoes photochemical reactions with biacetyl, with the product quantum yield influenced by the solvent. [, ]
Q6: Are there any safety concerns associated with handling 1,1-diethoxyethene?
A6: 1,1-Diethoxyethene is identified as a mutagen. [] It's crucial to handle it with caution, using appropriate safety equipment like gloves and working in a well-ventilated area.
Avertissement et informations sur les produits de recherche in vitro
Veuillez noter que tous les articles et informations sur les produits présentés sur BenchChem sont destinés uniquement à des fins informatives. Les produits disponibles à l'achat sur BenchChem sont spécifiquement conçus pour des études in vitro, qui sont réalisées en dehors des organismes vivants. Les études in vitro, dérivées du terme latin "in verre", impliquent des expériences réalisées dans des environnements de laboratoire contrôlés à l'aide de cellules ou de tissus. Il est important de noter que ces produits ne sont pas classés comme médicaments et n'ont pas reçu l'approbation de la FDA pour la prévention, le traitement ou la guérison de toute condition médicale, affection ou maladie. Nous devons souligner que toute forme d'introduction corporelle de ces produits chez les humains ou les animaux est strictement interdite par la loi. Il est essentiel de respecter ces directives pour assurer la conformité aux normes légales et éthiques en matière de recherche et d'expérimentation.