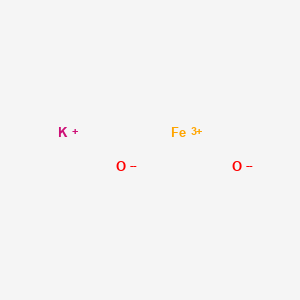
Ferrate de potassium
Vue d'ensemble
Description
Potassium ferrite is a compound with the chemical formula KFeO₂. It is a type of ferrite, which are ceramic materials composed primarily of iron oxide combined with other metal oxides. Potassium ferrite is known for its magnetic properties and has been studied for various applications, including biomedical uses, due to its potential in diagnostics, drug delivery, and treatment with magnetic hyperthermia .
Applications De Recherche Scientifique
Potassium ferrite has a wide range of scientific research applications, particularly in the fields of chemistry, biology, medicine, and industry. In chemistry, it is used as a catalyst for various organic reactions, providing a green synthesis method with reduced reaction times and fewer harmful byproducts . In biology and medicine, potassium ferrite nanoparticles are explored for their biocompatibility and potential in drug delivery systems and magnetic hyperthermia treatments . Industrially, potassium ferrite is used in the production of magnetic materials for electronic devices and transformers .
Mécanisme D'action
Target of Action
Potassium ferrate primarily targets a broad range of organic and inorganic compounds, including pollutants found in wastewater such as pesticides, pharmaceuticals, and drugs . It is also effective against microorganisms and viruses .
Mode of Action
Potassium ferrate interacts with its targets through oxidation, thanks to its extremely high redox potential . This interaction results in the decomposition of the target compounds . In the case of microorganisms and viruses, potassium ferrate can eliminate them .
Biochemical Pathways
The oxidation process facilitated by potassium ferrate disrupts the biochemical processes of targeted compounds. For instance, in the case of sludge cells, potassium ferrate facilitates the disruption of the cell and extracellular polymeric substances (EPS), increasing the proportion of biodegradable organics .
Result of Action
The action of potassium ferrate results in the formation of benign iron hydroxides . This is a significant advantage of using potassium ferrate, as these reaction products have minimal environmental load . In the context of wastewater treatment, the use of potassium ferrate leads to the effective removal of various pollutants .
Action Environment
The action, efficacy, and stability of potassium ferrate are influenced by environmental factors. For instance, potassium ferrate decomposes in contact with water, especially in acidic water . At high ph, aqueous solutions of potassium ferrate are stable . The reactivity of potassium ferrate also increases with temperature .
Analyse Biochimique
Biochemical Properties
Potassium ferrite plays a crucial role in biochemical reactions, particularly due to its magnetic properties. It interacts with various enzymes, proteins, and other biomolecules. For instance, potassium ferrite can bind to specific proteins, altering their conformation and activity. This interaction can influence enzymatic reactions, potentially enhancing or inhibiting the activity of certain enzymes. Additionally, potassium ferrite’s magnetic properties can be utilized to target specific biomolecules, facilitating their separation and analysis in biochemical assays .
Cellular Effects
Potassium ferrite has been shown to affect various types of cells and cellular processes. It can influence cell function by altering cell signaling pathways, gene expression, and cellular metabolism. For example, potassium ferrite can modulate the activity of signaling molecules, leading to changes in gene expression patterns. This can result in altered cellular metabolism, affecting processes such as energy production, cell growth, and apoptosis. Studies have demonstrated that potassium ferrite can induce cytotoxic effects at high concentrations, while lower concentrations may promote cell proliferation and differentiation .
Molecular Mechanism
The molecular mechanism of potassium ferrite involves its interaction with biomolecules at the molecular level. Potassium ferrite can bind to specific proteins, leading to conformational changes that affect their function. This binding can result in enzyme inhibition or activation, depending on the nature of the interaction. Additionally, potassium ferrite can influence gene expression by modulating the activity of transcription factors and other regulatory proteins. These molecular interactions contribute to the compound’s overall biochemical effects .
Temporal Effects in Laboratory Settings
In laboratory settings, the effects of potassium ferrite can change over time. The compound’s stability and degradation are critical factors that influence its long-term effects on cellular function. Potassium ferrite is sensitive to ambient conditions, and its interaction with moisture and carbon dioxide can lead to the formation of secondary phases. These changes can affect the compound’s biocompatibility and specific absorption rates. Long-term studies have shown that potassium ferrite can maintain its magnetic properties and biocompatibility over extended periods, although its cytotoxicity may vary depending on the concentration and exposure duration .
Dosage Effects in Animal Models
The effects of potassium ferrite vary with different dosages in animal models. At low doses, potassium ferrite has been shown to be biocompatible and can promote beneficial effects such as enhanced drug delivery and improved diagnostic imaging. At high doses, potassium ferrite can induce toxic effects, including oxidative stress, inflammation, and tissue damage. These adverse effects highlight the importance of determining the optimal dosage for therapeutic applications to minimize potential risks .
Metabolic Pathways
Potassium ferrite is involved in various metabolic pathways, interacting with enzymes and cofactors that regulate metabolic processes. The compound can influence metabolic flux and metabolite levels, affecting pathways such as glycolysis, the citric acid cycle, and oxidative phosphorylation. By modulating the activity of key enzymes, potassium ferrite can alter the production and utilization of energy within cells, impacting overall cellular metabolism .
Transport and Distribution
Within cells and tissues, potassium ferrite is transported and distributed through interactions with specific transporters and binding proteins. These interactions facilitate the compound’s localization and accumulation in target areas, enhancing its therapeutic efficacy. Potassium ferrite’s magnetic properties also enable its targeted delivery to specific tissues, improving its distribution and minimizing off-target effects .
Subcellular Localization
Potassium ferrite’s subcellular localization is influenced by targeting signals and post-translational modifications that direct it to specific compartments or organelles. The compound can accumulate in organelles such as mitochondria, lysosomes, and the endoplasmic reticulum, where it exerts its biochemical effects. This subcellular localization is critical for the compound’s activity and function, as it allows potassium ferrite to interact with specific biomolecules and influence cellular processes at the organelle level .
Méthodes De Préparation
Synthetic Routes and Reaction Conditions: Potassium ferrite can be synthesized using various methods, including the sol-gel process, hydrothermal synthesis, and combustion methods. One common method involves the proteic sol-gel route, where iron(III) nitrate and potassium nitrate are used as raw materials. The process involves mixing these nitrates with a proteic solution, such as powdered coconut water, followed by heat treatments at temperatures ranging from 350°C to 1300°C .
Industrial Production Methods: In industrial settings, potassium ferrite can be produced through solid-state reactions. This involves mixing iron(III) hydroxide with potassium nitrate and subjecting the mixture to high temperatures to form potassium ferrite. The process may also include washing the product with organic solvents to remove impurities .
Analyse Des Réactions Chimiques
Types of Reactions: Potassium ferrite undergoes various chemical reactions, including oxidation, reduction, and substitution reactions. For example, it can be used as a catalyst in oxidation reactions, where it helps in the degradation of organic dyes like methylene blue and crystal violet under visible light irradiation .
Common Reagents and Conditions: Common reagents used in reactions involving potassium ferrite include hydrogen peroxide for oxidation reactions and various organic solvents for purification. The reactions typically occur under controlled temperatures and atmospheric conditions to ensure the stability of the compound .
Major Products Formed: The major products formed from reactions involving potassium ferrite depend on the specific reaction conditions. For instance, in oxidation reactions, the primary products are often oxidized organic compounds and benign iron hydroxides .
Comparaison Avec Des Composés Similaires
Potassium ferrite can be compared with other ferrites such as barium ferrite, manganese ferrite, and zinc ferrite. While all these compounds share similar magnetic properties, potassium ferrite is unique due to its specific chemical composition and the resulting magnetic and catalytic properties. For instance, potassium ferrite has a narrower band gap compared to other ferrites, making it more efficient in photocatalytic applications . Similar compounds include:
- Barium ferrite (BaFe₁₂O₁₉)
- Manganese ferrite (MnFe₂O₄)
- Zinc ferrite (ZnFe₂O₄)
Each of these ferrites has distinct properties that make them suitable for different applications, but potassium ferrite stands out for its specific uses in green chemistry and biomedical applications .
Propriétés
IUPAC Name |
potassium;iron(3+);oxygen(2-) | |
---|---|---|
Source | PubChem | |
URL | https://pubchem.ncbi.nlm.nih.gov | |
Description | Data deposited in or computed by PubChem | |
InChI |
InChI=1S/Fe.K.2O/q+3;+1;2*-2 | |
Source | PubChem | |
URL | https://pubchem.ncbi.nlm.nih.gov | |
Description | Data deposited in or computed by PubChem | |
InChI Key |
UMPKMCDVBZFQOK-UHFFFAOYSA-N | |
Source | PubChem | |
URL | https://pubchem.ncbi.nlm.nih.gov | |
Description | Data deposited in or computed by PubChem | |
Canonical SMILES |
[O-2].[O-2].[K+].[Fe+3] | |
Source | PubChem | |
URL | https://pubchem.ncbi.nlm.nih.gov | |
Description | Data deposited in or computed by PubChem | |
Molecular Formula |
FeKO2 | |
Source | PubChem | |
URL | https://pubchem.ncbi.nlm.nih.gov | |
Description | Data deposited in or computed by PubChem | |
Molecular Weight |
126.94 g/mol | |
Source | PubChem | |
URL | https://pubchem.ncbi.nlm.nih.gov | |
Description | Data deposited in or computed by PubChem | |
Physical Description |
Other Solid | |
Record name | Iron potassium oxide (Fe11KO17) | |
Source | EPA Chemicals under the TSCA | |
URL | https://www.epa.gov/chemicals-under-tsca | |
Description | EPA Chemicals under the Toxic Substances Control Act (TSCA) collection contains information on chemicals and their regulations under TSCA, including non-confidential content from the TSCA Chemical Substance Inventory and Chemical Data Reporting. | |
CAS No. |
12160-44-0, 39469-86-8 | |
Record name | Iron potassium oxide (Fe11KO17) | |
Source | ChemIDplus | |
URL | https://pubchem.ncbi.nlm.nih.gov/substance/?source=chemidplus&sourceid=0012160440 | |
Description | ChemIDplus is a free, web search system that provides access to the structure and nomenclature authority files used for the identification of chemical substances cited in National Library of Medicine (NLM) databases, including the TOXNET system. | |
Record name | Potassium ferrate | |
Source | ChemIDplus | |
URL | https://pubchem.ncbi.nlm.nih.gov/substance/?source=chemidplus&sourceid=0039469868 | |
Description | ChemIDplus is a free, web search system that provides access to the structure and nomenclature authority files used for the identification of chemical substances cited in National Library of Medicine (NLM) databases, including the TOXNET system. | |
Record name | Iron potassium oxide (Fe11KO17) | |
Source | EPA Chemicals under the TSCA | |
URL | https://www.epa.gov/chemicals-under-tsca | |
Description | EPA Chemicals under the Toxic Substances Control Act (TSCA) collection contains information on chemicals and their regulations under TSCA, including non-confidential content from the TSCA Chemical Substance Inventory and Chemical Data Reporting. | |
Record name | potassium ferrite | |
Source | European Chemicals Agency (ECHA) | |
URL | https://echa.europa.eu/substance-information/-/substanceinfo/100.102.835 | |
Description | The European Chemicals Agency (ECHA) is an agency of the European Union which is the driving force among regulatory authorities in implementing the EU's groundbreaking chemicals legislation for the benefit of human health and the environment as well as for innovation and competitiveness. | |
Explanation | Use of the information, documents and data from the ECHA website is subject to the terms and conditions of this Legal Notice, and subject to other binding limitations provided for under applicable law, the information, documents and data made available on the ECHA website may be reproduced, distributed and/or used, totally or in part, for non-commercial purposes provided that ECHA is acknowledged as the source: "Source: European Chemicals Agency, http://echa.europa.eu/". Such acknowledgement must be included in each copy of the material. ECHA permits and encourages organisations and individuals to create links to the ECHA website under the following cumulative conditions: Links can only be made to webpages that provide a link to the Legal Notice page. | |
Q1: What spectroscopic data is available for characterizing Potassium ferrite?
A1: Several spectroscopic techniques are used to characterize potassium ferrite:
- X-ray diffraction (XRD): XRD reveals the crystalline structure of potassium ferrite, identifying phases like orthorhombic KFeO2 [, , , ] or the β″-Al2O3 structure [].
- Scanning Electron Microscopy (SEM) and Transmission Electron Microscopy (TEM): These techniques provide information about the morphology and size of potassium ferrite particles, revealing features like agglomeration, spherical morphology, and nanosheet formation [, , , ].
- Energy-dispersive X-ray spectroscopy (EDAX): EDAX confirms the elemental composition of potassium ferrite and can be used to verify the presence of dopants like silica [, ].
- Fourier Transform Infrared Spectrometer (FTIR): FTIR helps identify functional groups present in potassium ferrite and can confirm the presence of coatings like silica [].
- Mössbauer spectroscopy: This technique provides information about the oxidation state and coordination environment of iron atoms in potassium ferrite [, , ].
Q2: How does silica coating affect the properties of Potassium ferrite nanoparticles?
A3: Silica coating of Potassium ferrite nanoparticles reduces agglomeration, leading to a more uniform, spherical morphology [, ]. This coating also influences magnetic properties, decreasing magnetic saturation due to the presence of a magnetically dead layer of silica [, ].
Q3: What are the catalytic applications of Potassium ferrite?
A3: Potassium ferrite, particularly KFeO2, finds application in various catalytic processes:
- Dehydrogenation of Ethylbenzene: It acts as a key active phase in iron oxide catalysts used for converting ethylbenzene to styrene [, , , , ].
- Photo-Fenton Catalyst: K2Fe4O7, with its narrow band gap, exhibits efficient photocatalytic activity for degrading dyes like methylene blue and crystal violet in the presence of H2O2 under visible light [].
- Electrocatalytic Water Splitting: K2Fe4O7 nanocrystals demonstrate excellent electrocatalytic activity for both hydrogen evolution reaction (HER) and oxygen evolution reaction (OER), showing potential for large-scale hydrogen production [, ].
Q4: How does potassium influence the catalytic activity of iron oxide in dehydrogenation reactions?
A6: Potassium acts as a promoter in iron oxide catalysts, significantly enhancing the activity and selectivity for ethylbenzene dehydrogenation. It facilitates the formation of active potassium ferrite (KFeO2) and influences the surface chemical structure, creating active sites for the reaction [, , , ].
Q5: Have computational methods been used to study Potassium ferrite?
A8: Yes, computational methods like Density Functional Theory (DFT) have been employed to model the migration paths of ions in potassium ferrite structures []. These calculations help understand the ionic conductivity and factors influencing superionic behavior in these materials.
Q6: How does doping affect the properties of Potassium ferrite?
A6: Doping potassium ferrite with various elements can significantly alter its properties:
- Titanium (Ti) Doping: Improves ionic conductivity by widening migration channels in the crystal structure, but excessive doping can hinder conductivity [].
- Vanadium (V) Doping: Enhances the electrocatalytic activity of K2Fe4O7 for water splitting, improving both HER and OER performance [].
- Tin (Sn) Doping: Similar to vanadium doping, tin doping improves the electrocatalytic performance of potassium ferrite nanosheets for H2 production [].
- Divalent Metal Oxides: Doping with divalent metal oxides like MgO, ZnO, CaO, and SrO has been investigated for influencing the electrical conductivity and catalytic activity of potassium ferrites [, ].
Q7: How is potassium ferrite used in environmental remediation?
A10: Potassium ferrite, specifically in the form of KFeO2, can be used as an oxidizing agent for removing pollutants from water [, ]. Its strong oxidizing potential allows it to degrade organic contaminants and remove heavy metals, contributing to wastewater treatment.
Q8: What are the environmental concerns related to Potassium ferrite?
A8: While potassium ferrite itself might not pose significant environmental risks, the production and disposal processes need careful consideration. The use of harsh chemicals during synthesis and the potential release of metal ions during disposal require appropriate management to minimize environmental impact.
Q9: How is the presence and quantity of Potassium ferrite determined?
A9: Various analytical techniques are employed to identify and quantify Potassium ferrite:
Q10: What are the potential biomedical applications of Potassium ferrite?
A10: Potassium ferrite, especially in its nanoparticle form, has shown promise in several biomedical applications:
- Drug Delivery: The magnetic properties of potassium ferrite nanoparticles make them suitable for targeted drug delivery systems [].
- Hyperthermia Treatment: The ability of magnetic nanoparticles to generate heat upon exposure to an alternating magnetic field makes potassium ferrite a potential candidate for hyperthermia treatment of tumors [, ].
Q11: Is Potassium ferrite biocompatible?
A14: Biocompatibility of Potassium ferrite is dependent on factors like particle size, morphology, surface chemistry, and dosage. While some studies suggest biocompatibility at certain concentrations [, , ], thorough toxicological evaluations are crucial before clinical applications.
Avertissement et informations sur les produits de recherche in vitro
Veuillez noter que tous les articles et informations sur les produits présentés sur BenchChem sont destinés uniquement à des fins informatives. Les produits disponibles à l'achat sur BenchChem sont spécifiquement conçus pour des études in vitro, qui sont réalisées en dehors des organismes vivants. Les études in vitro, dérivées du terme latin "in verre", impliquent des expériences réalisées dans des environnements de laboratoire contrôlés à l'aide de cellules ou de tissus. Il est important de noter que ces produits ne sont pas classés comme médicaments et n'ont pas reçu l'approbation de la FDA pour la prévention, le traitement ou la guérison de toute condition médicale, affection ou maladie. Nous devons souligner que toute forme d'introduction corporelle de ces produits chez les humains ou les animaux est strictement interdite par la loi. Il est essentiel de respecter ces directives pour assurer la conformité aux normes légales et éthiques en matière de recherche et d'expérimentation.