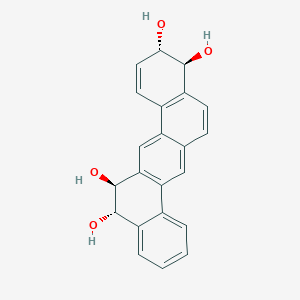
Amediplase
- Cliquez sur DEMANDE RAPIDE pour recevoir un devis de notre équipe d'experts.
- Avec des produits de qualité à un prix COMPÉTITIF, vous pouvez vous concentrer davantage sur votre recherche.
Vue d'ensemble
Description
Amediplase is a recombinant chimeric plasminogen activator. It consists of the kringle 2 domain from the A-chain of tissue plasminogen activator and the carboxy terminal region of pro-urokinase . This compound is primarily investigated for its potential use in treating myocardial infarction and thrombosis .
Méthodes De Préparation
Synthetic Routes and Reaction Conditions: Amediplase is produced using recombinant DNA techniques. The process involves the insertion of the gene encoding the kringle 2 domain of tissue plasminogen activator and the carboxy terminal region of pro-urokinase into a suitable expression vector. This vector is then introduced into Chinese hamster ovary cells, which express the recombinant protein .
Industrial Production Methods: The industrial production of this compound involves large-scale fermentation of the genetically modified Chinese hamster ovary cells. The recombinant protein is then purified using a series of chromatographic techniques to ensure homogeneity and activity .
Analyse Des Réactions Chimiques
Types of Reactions: Amediplase primarily undergoes proteolytic reactions, where it acts as a plasminogen activator. This involves the conversion of plasminogen to plasmin, a serine protease that degrades fibrin clots .
Common Reagents and Conditions: The activation of plasminogen by this compound requires the presence of fibrin, which acts as a cofactor. The reaction typically occurs under physiological conditions, with a pH of around 7.4 and a temperature of 37°C .
Major Products Formed: The primary product of the reaction catalyzed by this compound is plasmin, which subsequently degrades fibrin into soluble degradation products .
Applications De Recherche Scientifique
Amediplase has several scientific research applications, particularly in the fields of medicine and biology:
Mécanisme D'action
Comparaison Avec Des Composés Similaires
Propriétés
Numéro CAS |
151912-11-7 |
---|---|
Formule moléculaire |
C22H18O4 |
Poids moléculaire |
346.4 g/mol |
Nom IUPAC |
(3S,4S,12S,13S)-3,4,12,13-tetrahydronaphtho[1,2-b]phenanthrene-3,4,12,13-tetrol |
InChI |
InChI=1S/C22H18O4/c23-19-8-7-13-15(20(19)24)6-5-11-9-17-12-3-1-2-4-14(12)21(25)22(26)18(17)10-16(11)13/h1-10,19-26H/t19-,20-,21-,22-/m0/s1 |
Clé InChI |
YQINXCSNGCDFCQ-CMOCDZPBSA-N |
SMILES isomérique |
C1=CC=C2C(=C1)[C@@H]([C@H](C3=C2C=C4C=CC5=C(C4=C3)C=C[C@@H]([C@H]5O)O)O)O |
SMILES |
C1=CC=C2C(=C1)C(C(C3=C2C=C4C=CC5=C(C4=C3)C=CC(C5O)O)O)O |
SMILES canonique |
C1=CC=C2C(=C1)C(C(C3=C2C=C4C=CC5=C(C4=C3)C=CC(C5O)O)O)O |
151910-75-7 | |
Synonymes |
amediplase CGP 42935 K2tu-PA MEN 9036 |
Origine du produit |
United States |
Avertissement et informations sur les produits de recherche in vitro
Veuillez noter que tous les articles et informations sur les produits présentés sur BenchChem sont destinés uniquement à des fins informatives. Les produits disponibles à l'achat sur BenchChem sont spécifiquement conçus pour des études in vitro, qui sont réalisées en dehors des organismes vivants. Les études in vitro, dérivées du terme latin "in verre", impliquent des expériences réalisées dans des environnements de laboratoire contrôlés à l'aide de cellules ou de tissus. Il est important de noter que ces produits ne sont pas classés comme médicaments et n'ont pas reçu l'approbation de la FDA pour la prévention, le traitement ou la guérison de toute condition médicale, affection ou maladie. Nous devons souligner que toute forme d'introduction corporelle de ces produits chez les humains ou les animaux est strictement interdite par la loi. Il est essentiel de respecter ces directives pour assurer la conformité aux normes légales et éthiques en matière de recherche et d'expérimentation.