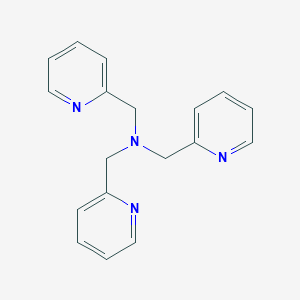
Tris(2-pyridylmethyl)amine
Vue d'ensemble
Description
Tris(2-pyridylmethyl)amine (TPA), with the molecular formula C₁₈H₁₈N₄, is a tripodal tetradentate ligand widely used in coordination chemistry and bioinorganic studies . Its structure features three pyridylmethyl arms bonded to a central amine, enabling strong yet flexible metal coordination. TPA forms stable complexes with transition metals such as Zn²⁺, Cu²⁺, and Fe²⁺/³⁺, making it invaluable in diverse applications:
- Biological Zinc Chelation: TPA rapidly intercepts mobile Zn²⁺ in live cells and brain slices with lower toxicity compared to TPEN (N,N,N',N'-tetrakis(2-pyridylmethyl)ethylenediamine) .
- Catalysis: TPA-derived copper complexes activate O₂ for peroxodicopper species, mimicking metalloenzymes .
- Polymerization: TPA (also abbreviated TPMA) acts as a ligand in atom transfer radical polymerization (ATRP), stabilizing Cu⁺ intermediates for controlled polymer synthesis .
- Sensing: ¹⁵N-labeled TPA derivatives enable MRI-based Zn²⁺ detection due to high selectivity and stability .
TPA’s synthesis involves reacting benzyl chlorides with acetaldehyde ammonia trimer under basic conditions, yielding tribenzylamine derivatives that are further functionalized .
Mécanisme D'action
Target of Action
Tris(2-pyridylmethyl)amine (TPMA or TPA) is a tertiary amine with three picolyl substituents . It is a tripodal ligand often used to simulate the coordination environment within some proteins . It is also used as a copper ligand in Atom Transfer Radical Polymerization (ATRP) .
Mode of Action
TPMA forms stable and catalytically active complexes with a wide variety of metals . It is known to exhibit anaerobic retro-Claisen type C–C bond cleavage reactivity . This reactivity exceeds that found in analogs supported by chelate ligands with fewer and/or weaker pyridyl interactions .
Biochemical Pathways
TPMA has been shown to have anticancer activity in osteosarcoma cell lines . It is introduced as a possible cationic species that could act as a counterpart for the decavanadate anion . The compound contains the previously reported vanadium (V) dioxido-tpma moieties, and the decavanadate anion is diprotonated . Studies on TPMA as an osteosarcoma antitumor agent are linked to higher miRNAs and proteins involved in several signaling pathways .
Pharmacokinetics
It is known to be a white solid that is soluble in polar organic solvents , which may influence its absorption, distribution, metabolism, and excretion (ADME) properties.
Result of Action
The result of TPMA’s action is largely dependent on its role as a ligand. In coordination chemistry, it forms complexes with metals, which can then participate in various reactions . In the context of anticancer activity, TPMA has been shown to exhibit activity against osteosarcoma cells .
Action Environment
The action of TPMA can be influenced by environmental factors. For example, its reactivity can be affected by the presence of oxygen, as it exhibits anaerobic retro-Claisen type C–C bond cleavage reactivity . Furthermore, its solubility in polar organic solvents suggests that the polarity of the environment could influence its action.
Analyse Biochimique
Biochemical Properties
Tris(2-pyridylmethyl)amine is known to interact with various biomolecules. For instance, it has been reported to exhibit anticancer activity in osteosarcoma cell lines . It has also been used as a ligand for Cu(II) 1,3-diketonate complexes, which exhibit anaerobic retro-Claisen type C–C bond cleavage reactivity .
Cellular Effects
The effects of this compound on cells are largely dependent on the specific biochemical context. In the context of cancer research, this compound has been found to have anticancer activity, potentially influencing cell signaling pathways, gene expression, and cellular metabolism .
Molecular Mechanism
The molecular mechanism of this compound is complex and multifaceted. It is known to interact with various biomolecules, potentially influencing enzyme activity and gene expression .
Temporal Effects in Laboratory Settings
Current studies have focused on its potential anticancer activity and its role as a ligand in Cu(II) 1,3-diketonate complexes .
Activité Biologique
Tris(2-pyridylmethyl)amine (TPA) is a tripodal ligand that has garnered significant attention in biological research due to its unique properties and potential therapeutic applications. This article delves into the biological activities of TPA, focusing on its anticancer effects, chelation properties, and its role as a radiopharmaceutical agent.
Anticancer Activity
Recent studies have highlighted TPA's potential as an anticancer agent, particularly against osteosarcoma cell lines.
- Cell Viability Studies : In experiments conducted on MG-63 and Saos-2 osteosarcoma cells, TPA demonstrated significant cytotoxicity. The half-maximal inhibitory concentration (IC50) values were reported as 50 µM for MG-63 cells and 91 µM for Saos-2 cells, indicating a dose-dependent decrease in cell viability .
- Mechanism of Action : The mechanism through which TPA exerts its anticancer effects appears to involve DNA interaction. Cyclic voltammetry studies indicated that TPA interacts with double-stranded DNA (dsDNA), which may contribute to its antitumor activity. Molecular docking studies further support this by suggesting specific binding modes between TPA and key biological targets related to osteosarcoma pathology .
- ADMET Profile : In silico predictions of the Absorption, Distribution, Metabolism, Excretion, and Toxicity (ADMET) properties suggest that TPA has a favorable pharmacokinetic profile with low toxicity risks, making it a promising candidate for further development as an anticancer drug .
Chelation Properties
TPA is recognized for its ability to chelate metal ions, particularly zinc. This property is crucial in various biological contexts:
- Zinc Chelation : TPA has been characterized as a membrane-permeable zinc chelator that intercepts biologically mobile zinc ions. Compared to other chelators like N,N,N',N'-tetrakis(2-pyridylmethyl)ethylenediamine (TPEN), TPA exhibits faster kinetics in chelating zinc in both live cells and brain slices. Its lower toxicity profile enhances its suitability for biological applications .
- Implications in Disease : The ability of TPA to modulate zinc levels has implications for diseases associated with zinc dysregulation, such as cancer and neurodegenerative disorders. By effectively chelating zinc, TPA can potentially influence cellular processes such as apoptosis and proliferation .
Radiopharmaceutical Applications
The development of radiolabeled derivatives of TPA has opened new avenues for imaging studies:
- PET Imaging : A novel probe, [^18F]-labeled TPA, has been developed to visualize zinc distribution in vivo using positron emission tomography (PET). This probe showed strong binding affinity for intracellular free Zn²⁺ ions and demonstrated high uptake in tissues such as the brain and bone during imaging studies .
- Synthesis and Stability : The synthesis of [^18F]-TPA was achieved with moderate yields, and stability tests indicated varying degrees of stability depending on the labeling position on the pyridyl group. This research highlights the potential of TPA-based probes for non-invasive imaging of zinc dynamics in various physiological and pathological states .
Summary of Key Findings
Study Aspect | Findings |
---|---|
Anticancer Activity | IC50 values of 50 µM (MG-63) and 91 µM (Saos-2); interacts with dsDNA |
Mechanism | DNA-binding; potential modulation of cancer pathways |
ADMET Profile | Favorable pharmacokinetics; low toxicity risk |
Zinc Chelation | Faster kinetics than TPEN; implications for cancer and neurodegeneration |
Radiopharmaceutical Use | Developed [^18F]-TPA probe; strong Zn²⁺ binding; useful for PET imaging |
Applications De Recherche Scientifique
Anticancer Activity
Mechanism of Action
TPMA has been investigated for its anticancer properties, particularly against osteosarcoma cell lines such as MG-63 and Saos-2. Studies have shown that TPMA exhibits cytotoxic effects, with IC50 values of 50 µM and 91 µM against these cell lines respectively. The mechanism involves interaction with DNA, as evidenced by cyclic voltammetry studies that indicate TPMA can bind to double-stranded DNA, potentially disrupting cancer cell proliferation .
Case Study
In one study, TPMA's interaction with DNA was characterized through molecular docking and cyclic voltammetry. The findings suggested that TPMA not only binds to DNA but also interacts with other biological targets relevant to osteosarcoma pathology. This dual action underscores its potential as a novel therapeutic agent in cancer treatment .
Zinc Chelation
Biological Significance
TPMA is recognized as a membrane-permeable zinc chelator. It intercepts mobile zinc ions within biological systems more effectively than other chelators like N,N,N',N'-tetrakis(2-pyridylmethyl)ethylenediamine (TPEN). This property is crucial for studying the role of zinc in various physiological processes and diseases, including neurodegenerative disorders and cancer .
Experimental Insights
Research has demonstrated that TPMA's chelation kinetics are superior to those of TPEN, making it a valuable tool for investigating zinc's biological functions. Its lower toxicity profile enhances its suitability for in vivo studies, further promoting its use in biomedical research .
Catalysis in Polymerization
Polymerization Applications
TPMA has also been utilized as a ligand in catalysis, particularly in atom transfer radical polymerization (ATRP). Recent studies indicated that TPMA could facilitate the polymerization of oligo(ethylene oxide) monomethyl ether methacrylate under visible light without the need for additional reagents. This method allows for the synthesis of polymers with low dispersity and high conversion rates .
Synthesis Process
In a notable experiment, TPMA was combined with copper bromide to initiate the polymerization process under blue or green light. The resulting polymers exhibited desirable characteristics such as low dispersity (Đ = 1.18–1.25), showcasing TPMA's effectiveness as a catalyst in polymer chemistry .
Summary of Applications
Application Area | Description | Key Findings |
---|---|---|
Anticancer Activity | Cytotoxic effects on osteosarcoma cell lines; interacts with DNA | IC50 values: 50 µM (MG-63), 91 µM (Saos-2); disrupts cancer cell proliferation |
Zinc Chelation | Membrane-permeable chelator intercepting mobile zinc | Faster kinetics than TPEN; lower toxicity; valuable for studying zinc biology |
Catalysis in Polymerization | Facilitates ATRP under visible light; synthesizes low-dispersity polymers | High conversion rates (>95%); effective as a catalyst |
Q & A
Q. Basic: What synthetic routes are available for TPA, and how can purity be optimized?
TPA is typically synthesized via a two-step reaction involving pyridine-2-carbaldehyde and 2-aminomethylpyridine. The first step forms an imine intermediate (N-(2-pyridylmethyl)-2-pyridylmethanimine), followed by reduction using agents like sodium cyanoborohydride under mild acidic conditions .
- Key Methodological Steps :
- Imine Formation : React pyridine-2-carbaldehyde with 2-aminomethylpyridine in anhydrous methanol at 50°C for 12 hours.
- Reduction : Add NaBH3CN in a 1:1 molar ratio under nitrogen atmosphere, followed by purification via column chromatography (e.g., Biotage® systems) to remove byproducts .
- Purity Optimization : Use HPLC with a C18 column (acetonitrile/water gradient) to confirm >98% purity.
Q. Basic: What analytical techniques are critical for characterizing TPA’s structure and coordination geometry?
- Single-Crystal XRD : Resolves bond lengths (e.g., N–N distances: 5.076–8.305 Å) and conformational details (e.g., π-π stacking in crystal lattices) .
- NMR Spectroscopy : 1H and 13C NMR confirm ligand proton environments and purity.
- UV-Vis and EPR : Monitor metal-ligand charge-transfer transitions and electronic states in complexes (e.g., Fe(II)/Fe(III) systems) .
Q. Advanced: How does TPA’s zinc-chelating efficiency compare to TPEN in biological systems?
TPA exhibits faster zinc-binding kinetics than TPEN due to its lower steric hindrance and weaker binding affinity (Kd ~10 nM vs. TPEN’s ~0.1 pM). This allows reversible zinc interception in live cells and brain slices with reduced cytotoxicity .
- Experimental Validation :
- Fluorescence Quenching : Use ZnAF-2 dye to track intracellular zinc dynamics.
- Kinetic Assays : Stopped-flow spectroscopy confirms TPA’s faster association rate (k_on ≈ 1.2 × 10^6 M⁻¹s⁻¹) .
Q. Advanced: How can TPA-based metal complexes be designed for catalytic oxidation reactions?
TPA’s tetradentate structure stabilizes high-valent metal centers. For example:
- Fe(IV)=O Complexes : Catalyze cyclohexane oxidation to adipic anhydride. Use [Ru(IV)(O)(TPA)(H2O)]²+ with H2O2 as an oxidant .
- Co(III) Complexes : Pair TPA with quinizarin ligands for DNA interaction studies. Structural optimization involves adjusting co-ligand electron-donating groups .
Q. Advanced: What mechanistic insights explain TPA’s role in oxidation reactions?
TPA stabilizes reactive intermediates via ligand-to-metal charge transfer (LMCT). For instance:
- Fe(IV)=O Formation : In CH3CN at -40°C, TPA-Fe(III) reacts with H2O2 to generate a transient Fe(IV)=O species, characterized by UV-Vis (λmax = 690 nm) and Mössbauer spectroscopy .
- Substrate Selectivity : Steric effects from pyridyl groups direct regioselective C–H bond activation .
Q. Advanced: How can computational methods model TPA’s metal-binding properties?
- DFT Calculations : Optimize geometries (e.g., B3LYP/6-31G*) to predict redox potentials and spin states (e.g., high-spin vs. low-spin Fe(II)) .
- MD Simulations : Analyze TPA’s membrane permeability by modeling lipid bilayer interactions .
Q. Advanced: What kinetic parameters define TPA’s biological zinc chelation?
- Association Rate (k_on) : 1.2 × 10^6 M⁻¹s⁻¹ (measured via stopped-flow in PBS buffer, pH 7.4) .
- Dissociation Rate (k_off) : 0.15 s⁻¹, enabling transient zinc buffering in neurons .
Q. Advanced: How can TPA derivatives enhance catalytic or biomedical performance?
Comparaison Avec Des Composés Similaires
TPA’s properties are best contextualized against structurally related ligands. Below is a detailed comparison:
Table 1: Comparative Analysis of TPA and Key Analogues
Key Findings from Comparative Studies
TPA vs. TPEN :
- TPA’s smaller size and lower pKa (~6.5 vs. TPEN’s ~1.7) enable faster Zn²⁺ binding kinetics in biological systems. Its weaker affinity (log K ~10.8 for Zn²⁺) reduces cellular toxicity, making it preferable for live-cell imaging .
TPA vs. TQA: Replacing pyridyl with quinolyl groups (TQA) red-shifts fluorescence emission, improving Zn²⁺ probe sensitivity. However, TQA’s bulkier structure may reduce membrane permeability compared to TPA .
TPA vs. BPMEN :
- BPMEN’s ethanediamine backbone creates a more rigid geometry, favoring trans-μ-1,2-peroxo dicopper species, while TPA derivatives form hydrogen-bonded peroxo complexes .
TPA vs. Me₆TREN :
- Me₆TREN’s tertiary amines provide stronger Cu⁺ stabilization in ATRP, but TPA’s pyridyl groups offer better redox stability in aqueous media .
TPA in NO Generation: TPA outperforms BMPA-Pr and BEPA-Pr in electrochemically modulated NO release, attributed to its balanced Cu²⁺ affinity and resistance to oxidative degradation .
Electronic and Steric Tuning of TPA Derivatives
- 6-Substituted TPA Ligands: Adding electron-donating (e.g., -OMe) or withdrawing (e.g., -CF₃) groups at the pyridyl 6-position modulates Cu-O₂ reactivity.
- ¹⁵N-Labeled TPA : Isotopic labeling enables hyperpolarized MRI detection of Zn²⁺, leveraging TPA’s high selectivity over competing ions like Ca²⁺ or Mg²⁺ .
Propriétés
IUPAC Name |
1-pyridin-2-yl-N,N-bis(pyridin-2-ylmethyl)methanamine | |
---|---|---|
Source | PubChem | |
URL | https://pubchem.ncbi.nlm.nih.gov | |
Description | Data deposited in or computed by PubChem | |
InChI |
InChI=1S/C18H18N4/c1-4-10-19-16(7-1)13-22(14-17-8-2-5-11-20-17)15-18-9-3-6-12-21-18/h1-12H,13-15H2 | |
Source | PubChem | |
URL | https://pubchem.ncbi.nlm.nih.gov | |
Description | Data deposited in or computed by PubChem | |
InChI Key |
VGUWFGWZSVLROP-UHFFFAOYSA-N | |
Source | PubChem | |
URL | https://pubchem.ncbi.nlm.nih.gov | |
Description | Data deposited in or computed by PubChem | |
Canonical SMILES |
C1=CC=NC(=C1)CN(CC2=CC=CC=N2)CC3=CC=CC=N3 | |
Source | PubChem | |
URL | https://pubchem.ncbi.nlm.nih.gov | |
Description | Data deposited in or computed by PubChem | |
Molecular Formula |
C18H18N4 | |
Source | PubChem | |
URL | https://pubchem.ncbi.nlm.nih.gov | |
Description | Data deposited in or computed by PubChem | |
DSSTOX Substance ID |
DTXSID70327519 | |
Record name | Tris(2-pyridylmethyl)amine | |
Source | EPA DSSTox | |
URL | https://comptox.epa.gov/dashboard/DTXSID70327519 | |
Description | DSSTox provides a high quality public chemistry resource for supporting improved predictive toxicology. | |
Molecular Weight |
290.4 g/mol | |
Source | PubChem | |
URL | https://pubchem.ncbi.nlm.nih.gov | |
Description | Data deposited in or computed by PubChem | |
CAS No. |
16858-01-8 | |
Record name | Tris(2-pyridylmethyl)amine | |
Source | CAS Common Chemistry | |
URL | https://commonchemistry.cas.org/detail?cas_rn=16858-01-8 | |
Description | CAS Common Chemistry is an open community resource for accessing chemical information. Nearly 500,000 chemical substances from CAS REGISTRY cover areas of community interest, including common and frequently regulated chemicals, and those relevant to high school and undergraduate chemistry classes. This chemical information, curated by our expert scientists, is provided in alignment with our mission as a division of the American Chemical Society. | |
Explanation | The data from CAS Common Chemistry is provided under a CC-BY-NC 4.0 license, unless otherwise stated. | |
Record name | Tris(2-pyridylmethyl)amine | |
Source | ChemIDplus | |
URL | https://pubchem.ncbi.nlm.nih.gov/substance/?source=chemidplus&sourceid=0016858018 | |
Description | ChemIDplus is a free, web search system that provides access to the structure and nomenclature authority files used for the identification of chemical substances cited in National Library of Medicine (NLM) databases, including the TOXNET system. | |
Record name | Tris(2-pyridylmethyl)amine | |
Source | EPA DSSTox | |
URL | https://comptox.epa.gov/dashboard/DTXSID70327519 | |
Description | DSSTox provides a high quality public chemistry resource for supporting improved predictive toxicology. | |
Record name | TRIS(2-PYRIDYLMETHYL)AMINE | |
Source | FDA Global Substance Registration System (GSRS) | |
URL | https://gsrs.ncats.nih.gov/ginas/app/beta/substances/V4CE3X87Z3 | |
Description | The FDA Global Substance Registration System (GSRS) enables the efficient and accurate exchange of information on what substances are in regulated products. Instead of relying on names, which vary across regulatory domains, countries, and regions, the GSRS knowledge base makes it possible for substances to be defined by standardized, scientific descriptions. | |
Explanation | Unless otherwise noted, the contents of the FDA website (www.fda.gov), both text and graphics, are not copyrighted. They are in the public domain and may be republished, reprinted and otherwise used freely by anyone without the need to obtain permission from FDA. Credit to the U.S. Food and Drug Administration as the source is appreciated but not required. | |
Synthesis routes and methods
Procedure details
Retrosynthesis Analysis
AI-Powered Synthesis Planning: Our tool employs the Template_relevance Pistachio, Template_relevance Bkms_metabolic, Template_relevance Pistachio_ringbreaker, Template_relevance Reaxys, Template_relevance Reaxys_biocatalysis model, leveraging a vast database of chemical reactions to predict feasible synthetic routes.
One-Step Synthesis Focus: Specifically designed for one-step synthesis, it provides concise and direct routes for your target compounds, streamlining the synthesis process.
Accurate Predictions: Utilizing the extensive PISTACHIO, BKMS_METABOLIC, PISTACHIO_RINGBREAKER, REAXYS, REAXYS_BIOCATALYSIS database, our tool offers high-accuracy predictions, reflecting the latest in chemical research and data.
Strategy Settings
Precursor scoring | Relevance Heuristic |
---|---|
Min. plausibility | 0.01 |
Model | Template_relevance |
Template Set | Pistachio/Bkms_metabolic/Pistachio_ringbreaker/Reaxys/Reaxys_biocatalysis |
Top-N result to add to graph | 6 |
Feasible Synthetic Routes
Avertissement et informations sur les produits de recherche in vitro
Veuillez noter que tous les articles et informations sur les produits présentés sur BenchChem sont destinés uniquement à des fins informatives. Les produits disponibles à l'achat sur BenchChem sont spécifiquement conçus pour des études in vitro, qui sont réalisées en dehors des organismes vivants. Les études in vitro, dérivées du terme latin "in verre", impliquent des expériences réalisées dans des environnements de laboratoire contrôlés à l'aide de cellules ou de tissus. Il est important de noter que ces produits ne sont pas classés comme médicaments et n'ont pas reçu l'approbation de la FDA pour la prévention, le traitement ou la guérison de toute condition médicale, affection ou maladie. Nous devons souligner que toute forme d'introduction corporelle de ces produits chez les humains ou les animaux est strictement interdite par la loi. Il est essentiel de respecter ces directives pour assurer la conformité aux normes légales et éthiques en matière de recherche et d'expérimentation.