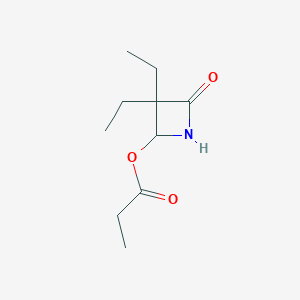
3,3-Diethyl-4-oxoazetidin-2-yl propionate
- Cliquez sur DEMANDE RAPIDE pour recevoir un devis de notre équipe d'experts.
- Avec des produits de qualité à un prix COMPÉTITIF, vous pouvez vous concentrer davantage sur votre recherche.
Vue d'ensemble
Description
Méthodes De Préparation
The synthesis of 3,3-Diethyl-4-oxoazetidin-2-yl propionate involves several steps. One common method includes the reaction of diethylamine with ethyl chloroformate to form an intermediate, which is then cyclized to produce the azetidinone ring . The final step involves esterification with propionic acid to yield the desired compound . Industrial production methods typically involve similar steps but are optimized for larger scale production and higher yields .
Analyse Des Réactions Chimiques
3,3-Diethyl-4-oxoazetidin-2-yl propionate undergoes various chemical reactions, including:
Applications De Recherche Scientifique
3,3-Diethyl-4-oxoazetidin-2-yl propionate has several applications in scientific research:
Mécanisme D'action
The mechanism of action of 3,3-Diethyl-4-oxoazetidin-2-yl propionate involves its interaction with specific molecular targets. The compound can inhibit certain enzymes or receptors, leading to various biological effects . The exact pathways and targets are still under investigation, but it is believed to involve modulation of cellular signaling pathways .
Comparaison Avec Des Composés Similaires
3,3-Diethyl-4-oxoazetidin-2-yl propionate can be compared with other azetidinone derivatives, such as:
3,3-Dimethyl-4-oxoazetidin-2-yl propionate: Similar structure but with methyl groups instead of ethyl groups, leading to different chemical and biological properties.
3,3-Diethyl-4-oxoazetidin-2-yl acetate: Similar structure but with an acetate group instead of a propionate group, affecting its reactivity and applications.
Propriétés
IUPAC Name |
(3,3-diethyl-4-oxoazetidin-2-yl) propanoate |
Source
|
---|---|---|
Source | PubChem | |
URL | https://pubchem.ncbi.nlm.nih.gov | |
Description | Data deposited in or computed by PubChem | |
InChI |
InChI=1S/C10H17NO3/c1-4-7(12)14-9-10(5-2,6-3)8(13)11-9/h9H,4-6H2,1-3H3,(H,11,13) |
Source
|
Source | PubChem | |
URL | https://pubchem.ncbi.nlm.nih.gov | |
Description | Data deposited in or computed by PubChem | |
InChI Key |
JEUBRNMPSDDATF-UHFFFAOYSA-N |
Source
|
Source | PubChem | |
URL | https://pubchem.ncbi.nlm.nih.gov | |
Description | Data deposited in or computed by PubChem | |
Canonical SMILES |
CCC(=O)OC1C(C(=O)N1)(CC)CC |
Source
|
Source | PubChem | |
URL | https://pubchem.ncbi.nlm.nih.gov | |
Description | Data deposited in or computed by PubChem | |
Molecular Formula |
C10H17NO3 |
Source
|
Source | PubChem | |
URL | https://pubchem.ncbi.nlm.nih.gov | |
Description | Data deposited in or computed by PubChem | |
Molecular Weight |
199.25 g/mol |
Source
|
Source | PubChem | |
URL | https://pubchem.ncbi.nlm.nih.gov | |
Description | Data deposited in or computed by PubChem | |
Avertissement et informations sur les produits de recherche in vitro
Veuillez noter que tous les articles et informations sur les produits présentés sur BenchChem sont destinés uniquement à des fins informatives. Les produits disponibles à l'achat sur BenchChem sont spécifiquement conçus pour des études in vitro, qui sont réalisées en dehors des organismes vivants. Les études in vitro, dérivées du terme latin "in verre", impliquent des expériences réalisées dans des environnements de laboratoire contrôlés à l'aide de cellules ou de tissus. Il est important de noter que ces produits ne sont pas classés comme médicaments et n'ont pas reçu l'approbation de la FDA pour la prévention, le traitement ou la guérison de toute condition médicale, affection ou maladie. Nous devons souligner que toute forme d'introduction corporelle de ces produits chez les humains ou les animaux est strictement interdite par la loi. Il est essentiel de respecter ces directives pour assurer la conformité aux normes légales et éthiques en matière de recherche et d'expérimentation.