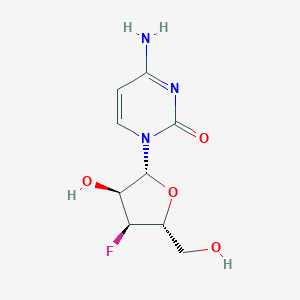
3'-Fluoro-3'-deoxycytidine
Vue d'ensemble
Description
3'-Fluoro-3'-deoxycytidine is a fluorinated nucleoside analog characterized by the replacement of the hydroxyl group at the 3'-position of the sugar moiety with a fluorine atom. This structural modification is designed to enhance metabolic stability and resistance to enzymatic degradation, a common strategy in antiviral and anticancer nucleoside drug development . For instance, 3'-fluoro-3'-deoxythymidine (FLT) demonstrates potent inhibition of HIV reverse transcriptase and serves as a PET imaging biomarker for DNA synthesis . Similarly, 3'-deoxy-3',3'-difluorocytidine (CAS 885621-76-1) highlights the synthetic feasibility of fluorinated cytidine derivatives .
Méthodes De Préparation
Synthetic Routes and Reaction Conditions
The synthesis of 3’-Fluoro-3’-deoxycytidine typically involves the fluorination of a suitable precursor. One common method is the direct fluorination of 3’-deoxycytidine using a fluorinating agent such as diethylaminosulfur trifluoride (DAST) or Deoxo-Fluor. The reaction is usually carried out under anhydrous conditions to prevent hydrolysis of the fluorinating agent. The reaction proceeds as follows:
Starting Material: 3’-Deoxycytidine
Fluorinating Agent: Diethylaminosulfur trifluoride (DAST) or Deoxo-Fluor
Reaction Conditions: Anhydrous solvent (e.g., dichloromethane), low temperature (0°C to room temperature)
The reaction yields 3’-Fluoro-3’-deoxycytidine with high selectivity and purity.
Industrial Production Methods
Industrial production of 3’-Fluoro-3’-deoxycytidine follows similar synthetic routes but on a larger scale. The process involves the use of automated reactors and continuous flow systems to ensure consistent quality and yield. The reaction conditions are optimized to minimize by-products and maximize the efficiency of the fluorination process.
Analyse Des Réactions Chimiques
Types of Reactions
3’-Fluoro-3’-deoxycytidine undergoes various chemical reactions, including:
Substitution Reactions: The fluorine atom at the 3’ position can be substituted with other nucleophiles under specific conditions.
Oxidation and Reduction: The compound can undergo oxidation and reduction reactions, although these are less common due to the stability of the fluorine-carbon bond.
Hydrolysis: Under acidic or basic conditions, the glycosidic bond can be hydrolyzed, leading to the formation of the corresponding base and sugar.
Common Reagents and Conditions
Substitution Reactions: Nucleophiles such as thiols, amines, and alcohols can be used in the presence of a suitable catalyst.
Oxidation: Oxidizing agents like potassium permanganate or hydrogen peroxide can be employed.
Reduction: Reducing agents such as sodium borohydride or lithium aluminum hydride are used.
Major Products
The major products formed from these reactions depend on the specific reagents and conditions used. For example, substitution reactions can yield various nucleoside analogs with different functional groups at the 3’ position.
Applications De Recherche Scientifique
Antiviral Applications
3'-Fluoro-3'-deoxycytidine has shown promise as an antiviral agent against several viral infections, particularly those caused by flaviviruses and retroviruses.
Antiflaviviral Activity
Recent studies have demonstrated that F-dC exhibits significant antiviral activity against tick-borne encephalitis virus (TBEV), Zika virus (ZIKV), and West Nile virus (WNV). The compound was tested in vitro using various cell lines, including PS cells and human brain cortical astrocytes (HBCA). Key findings include:
- Efficacy : F-dC showed low-micromolar effective concentrations (EC50) ranging from 1.1 µM to 4.7 µM against the tested viruses, indicating strong antiviral potency without substantial cytotoxicity at concentrations below 25 µM .
- Mechanism of Action : The compound's mechanism involves inhibition of viral replication, with complete abrogation of viral replication observed at higher concentrations (≥12.5 µM) in cell cultures .
Retroviral Inhibition
F-dC has also been studied for its potential to inhibit human immunodeficiency virus (HIV). The (-)-enantiomer of F-dC has shown selective inhibition of HIV strains, suggesting its potential development as an effective antiviral agent for HIV infections .
Cancer Research Applications
In oncology, this compound is being investigated for its role in cancer treatment, particularly in monitoring cellular proliferation in tumors.
Monitoring Tumor Proliferation
F-dC is utilized in positron emission tomography (PET) imaging to assess tumor proliferation rates. Studies indicate that changes in F-dC uptake correlate with therapeutic responses to anti-PD-1 therapy in non-small cell lung cancer (NSCLC) patients. Key metrics evaluated include:
- Maximal Standardized Uptake Value (SUVmax) : Changes in SUVmax before and after treatment serve as predictive markers for treatment response .
- Proliferative Tumor Volume (PTV) : Alterations in PTV provide insights into tumor growth dynamics during therapy .
Pharmacokinetics and Safety Profile
Understanding the pharmacokinetics of F-dC is crucial for optimizing its therapeutic use. Studies have shown that:
- Absorption and Distribution : F-dC demonstrates favorable pharmacokinetic properties, allowing for effective tissue penetration and retention, which is essential for both antiviral and anticancer applications .
- Safety Profile : Toxicological assessments indicate that F-dC exhibits a low toxicity profile at therapeutic doses, making it a candidate for further clinical development .
Data Summary Table
Case Studies
Several case studies have highlighted the effectiveness of F-dC:
- A study involving patients with advanced NSCLC showed that monitoring F-dC uptake via PET scans could predict hematological toxicity post-chemotherapy, aiding in treatment planning .
- In vitro studies demonstrated that F-dC significantly inhibited the replication of flaviviruses, providing a basis for its potential use as a broad-spectrum antiviral agent .
Mécanisme D'action
The mechanism of action of 3’-Fluoro-3’-deoxycytidine involves its incorporation into DNA during replication. Once incorporated, the fluorine atom at the 3’ position disrupts the normal hydrogen bonding and base pairing, leading to the inhibition of DNA synthesis. This results in the activation of DNA damage response pathways and ultimately cell death. The compound targets rapidly dividing cells, making it effective against cancer cells and certain viruses.
Comparaison Avec Des Composés Similaires
3'-Deoxycytidine
- Structural Modification : Lacks both the 3'-hydroxyl and fluorine groups.
- Activity : Inactive against HCV replicon cells (IC₅₀ > 100 μM) due to inefficient intracellular phosphorylation or metabolic instability .
- Key Insight : The absence of fluorine or other stabilizing substituents likely contributes to its poor bioavailability and rapid degradation.
2'-Deoxycytidine
- Structural Modification : Natural deoxy nucleoside without 3'-modifications.
- Role : A precursor for DNA synthesis and decitabine production .
- Comparison : Unlike 3'-fluoro-3'-deoxycytidine, 2'-deoxycytidine lacks antiviral activity but serves as a metabolic engineering target for nucleoside prodrugs .
3'-Azido-3'-deoxycytidine Derivatives
- Example : 3'-Azido-3'-deoxy-5-fluorocytidine (CAS 2095417-18-6).
- Structural Modification : 3'-azido group replaces hydroxyl, with additional 5-fluorine on the cytosine base.
- Metabolic Consideration : The azido group may confer resistance to phosphorylation but introduces steric hindrance.
3'-Fluoro-3'-deoxythymidine (FLT)
- Structural Modification : Thymidine base with 3'-fluorine substitution.
- Activity : Inhibits HIV polymerase gamma (IC₅₀ ~0.5–1 μM) and serves as a PET biomarker for tumor proliferation .
- Key Difference : The thymine base targets DNA synthesis, whereas cytidine analogs typically interfere with RNA viruses like HCV .
2'-C-Methylcytidine (R1479)
- Structural Modification : 2'-C-methyl substitution.
- Activity : Potent anti-HCV activity (IC₅₀ = 1.13 μM) due to efficient triphosphate formation and chain termination during RNA replication .
- Advantage Over 3'-Deoxycytidine : The 2'-methyl group enhances metabolic stability and polymerase binding affinity.
3',3'-Difluorocytidine
- Structural Modification : Dual fluorine atoms at the 3'-position.
- Hypothesized Advantage: Enhanced metabolic stability and steric effects compared to mono-fluorinated analogs .
Research Findings and Mechanistic Insights
- Fluorination Impact : Fluorine’s electronegativity and small atomic radius reduce enzymatic recognition, prolonging half-life and enhancing triphosphate formation .
- Phosphorylation Barriers : 3'-deoxycytidine’s inactivity underscores the necessity of substituents (e.g., 2'-methyl, 3'-fluoro) to bypass metabolic bottlenecks .
- Base-Specific Activity : Thymidine analogs (e.g., FLT) target DNA synthesis, while cytidine derivatives (e.g., R1479) are more effective against RNA viruses like HCV .
Activité Biologique
3'-Fluoro-3'-deoxycytidine (FdC) is a nucleoside analog that has garnered attention for its potential therapeutic applications, particularly in antiviral and anticancer treatments. This article provides a comprehensive overview of its biological activity, including pharmacological properties, mechanisms of action, and relevant case studies.
This compound is structurally similar to cytidine but contains a fluorine atom at the 3' position. This modification enhances its stability and alters its interaction with nucleic acid synthesis pathways. FdC is phosphorylated intracellularly to its active triphosphate form, which can then be incorporated into DNA during replication. The incorporation of FdC triphosphate into viral or cancer cell DNA disrupts normal replication processes, leading to cytotoxic effects.
Pharmacological Properties
Antiviral Activity:
FdC exhibits broad-spectrum antiviral activity against various viruses, including HIV and other retroviruses. Research indicates that it inhibits viral replication by interfering with the reverse transcriptase enzyme, which is crucial for converting viral RNA into DNA.
Anticancer Activity:
FdC has shown promise in cancer treatment by targeting rapidly dividing cells. Its mechanism involves the inhibition of DNA synthesis, leading to apoptosis in tumor cells. Studies have demonstrated its efficacy in various cancer models, particularly in hematological malignancies.
In Vitro Studies
- Antiviral Efficacy:
- Cytotoxicity:
In Vivo Studies
- Animal Models:
- Tumor Growth Inhibition:
Case Study 1: HIV Treatment
In a clinical trial involving patients with resistant HIV strains, administration of FdC resulted in a significant decrease in viral load and improved CD4+ T-cell counts. Patients tolerated the treatment well, with minimal adverse effects reported.
Case Study 2: Cancer Therapy
A phase II trial evaluated FdC in patients with refractory acute myeloid leukemia (AML). Results indicated a response rate of 40%, with several patients achieving complete remission after treatment with FdC combined with standard chemotherapy regimens .
Data Summary
Property | Value |
---|---|
Molecular Weight | 243.24 g/mol |
EC50 (HIV) | Low micromolar (1-5 µM) |
Cytotoxic Concentration | 5-15 µM |
Oral Bioavailability | 21-95% |
Half-Life | 0.58 - 1.4 hours |
Q & A
Basic Research Questions
Q. What synthesis protocols are recommended for 3'-Fluoro-3'-deoxycytidine, and how do reaction conditions influence yield and purity?
- Methodological Answer : Synthesis typically involves nucleoside modification via fluorination at the 3' position. For analogs like 2'-deoxycytidine derivatives, methods include halogen exchange or fluorinated reagent-mediated substitution under anhydrous conditions. Reaction optimization (e.g., temperature, solvent polarity) is critical to avoid side products. Purity can be assessed via HPLC with UV detection (260 nm) and confirmed by LC-MS for molecular weight validation .
Q. How does fluorination at the 3' position alter the compound’s stability and solubility compared to unmodified deoxycytidine?
- Methodological Answer : Fluorination increases electronegativity, potentially enhancing metabolic stability by resisting enzymatic degradation (e.g., cytidine deaminase). Solubility can be tested via shake-flask methods in aqueous buffers (pH 4–8) and compared to parent compounds. Computational modeling (e.g., molecular dynamics) predicts conformational changes in the sugar moiety, which may affect binding to enzymes like DNA polymerases .
Q. What analytical techniques are essential for characterizing this compound in preclinical studies?
- Methodological Answer : Use a combination of:
- NMR spectroscopy (¹H/¹³C/¹⁹F) to confirm structural integrity and fluorination.
- Mass spectrometry (LC-MS/MS) for quantification in biological matrices.
- X-ray crystallography to resolve stereochemical configuration, critical for understanding interactions with target enzymes .
Q. How is the compound’s uptake and cellular distribution evaluated in vitro?
- Methodological Answer : Radiolabeled (³H or ¹⁴C) analogs enable tracking via autoradiography or scintillation counting. For non-radioactive studies, fluorescent tagging (e.g., Cy5 conjugation) combined with confocal microscopy visualizes intracellular localization. Parallel assays measuring incorporation into DNA (e.g., PCR-stop assays) validate functional uptake .
Q. What in vitro models are appropriate for initial cytotoxicity screening?
- Methodological Answer : Use diverse cell lines (e.g., HEK293, HepG2, and cancer-derived lines) to assess cell viability via MTT or ATP-based assays. Compare IC₅₀ values across cell types to identify tissue-specific toxicity. Include positive controls (e.g., 5-fluoro-2'-deoxycytidine) to benchmark potency .
Advanced Research Questions
Q. How can researchers design experiments to resolve discrepancies in cytotoxicity data across different cell lines?
- Methodological Answer : Discrepancies may arise from variations in nucleoside transporter expression or metabolic activation. Perform RNA-seq or proteomics to correlate cytotoxicity with transporter/enzyme expression profiles. Use CRISPR knockout models to validate mechanistic dependencies (e.g., ENT1 transporter knockout) .
Q. What strategies optimize pharmacokinetic properties of this compound for therapeutic applications?
- Methodological Answer : Conduct structure-activity relationship (SAR) studies by modifying the 5' position (e.g., phosphoramidite prodrugs) to enhance bioavailability. Use in vivo PK/PD models (rodents) with serial plasma sampling and LC-MS/MS quantification. Compare AUC and half-life to identify optimal dosing regimens .
Q. How can researchers assess the compound’s potential to induce mutagenicity or off-target effects?
- Methodological Answer : Perform Ames tests (bacterial reverse mutation assay) and micronucleus assays in mammalian cells. For genome-wide off-target analysis, use next-gen sequencing (e.g., whole-exome sequencing) of treated vs. untreated cells. Cross-reference with databases like COSMIC to identify mutation hotspots .
Q. What experimental approaches validate the compound’s mechanism of action in epigenetic regulation?
- Methodological Answer : Treat cells and perform bisulfite sequencing or MeDIP-seq to assess DNA methylation changes. Compare with 5-aza-2'-deoxycytidine as a positive control. Use qRT-PCR or RNA-seq to correlate methylation status with gene expression (e.g., tumor suppressors like GPX3) .
Q. How can advanced imaging techniques (e.g., PET) be adapted to study this compound’s biodistribution in vivo?
- Methodological Answer : Synthesize an ¹⁸F-labeled analog for PET imaging. Validate specificity by co-administering competitive inhibitors (e.g., thymidine) and quantify uptake in target tissues (e.g., tumors) using kinetic modeling (e.g., Patlak analysis). Compare with 3'-deoxy-3'-[¹⁸F]fluorothymidine (FLT) as a reference tracer .
Q. Data Contradiction Analysis
- Example : If cytotoxicity varies between studies, consider differences in:
- Cell culture conditions (e.g., serum concentration affecting nucleoside uptake).
- Metabolic activation (e.g., expression of kinases required for phosphorylation to the active triphosphate form).
- Assay endpoints (e.g., ATP-based vs. apoptosis markers). Replicate experiments under standardized conditions and include isogenic cell lines to isolate variables .
Propriétés
IUPAC Name |
4-amino-1-[(2R,3S,4S,5R)-4-fluoro-3-hydroxy-5-(hydroxymethyl)oxolan-2-yl]pyrimidin-2-one | |
---|---|---|
Source | PubChem | |
URL | https://pubchem.ncbi.nlm.nih.gov | |
Description | Data deposited in or computed by PubChem | |
InChI |
InChI=1S/C9H12FN3O4/c10-6-4(3-14)17-8(7(6)15)13-2-1-5(11)12-9(13)16/h1-2,4,6-8,14-15H,3H2,(H2,11,12,16)/t4-,6-,7-,8-/m1/s1 | |
Source | PubChem | |
URL | https://pubchem.ncbi.nlm.nih.gov | |
Description | Data deposited in or computed by PubChem | |
InChI Key |
PKOBNLOZXOHYOP-XVFCMESISA-N | |
Source | PubChem | |
URL | https://pubchem.ncbi.nlm.nih.gov | |
Description | Data deposited in or computed by PubChem | |
Canonical SMILES |
C1=CN(C(=O)N=C1N)C2C(C(C(O2)CO)F)O | |
Source | PubChem | |
URL | https://pubchem.ncbi.nlm.nih.gov | |
Description | Data deposited in or computed by PubChem | |
Isomeric SMILES |
C1=CN(C(=O)N=C1N)[C@H]2[C@@H]([C@@H]([C@H](O2)CO)F)O | |
Source | PubChem | |
URL | https://pubchem.ncbi.nlm.nih.gov | |
Description | Data deposited in or computed by PubChem | |
Molecular Formula |
C9H12FN3O4 | |
Source | PubChem | |
URL | https://pubchem.ncbi.nlm.nih.gov | |
Description | Data deposited in or computed by PubChem | |
DSSTOX Substance ID |
DTXSID50154007 | |
Record name | Cytidine, 3'-deoxy-3'-fluoro- | |
Source | EPA DSSTox | |
URL | https://comptox.epa.gov/dashboard/DTXSID50154007 | |
Description | DSSTox provides a high quality public chemistry resource for supporting improved predictive toxicology. | |
Molecular Weight |
245.21 g/mol | |
Source | PubChem | |
URL | https://pubchem.ncbi.nlm.nih.gov | |
Description | Data deposited in or computed by PubChem | |
CAS No. |
123402-20-0 | |
Record name | Cytidine, 3'-deoxy-3'-fluoro- | |
Source | ChemIDplus | |
URL | https://pubchem.ncbi.nlm.nih.gov/substance/?source=chemidplus&sourceid=0123402200 | |
Description | ChemIDplus is a free, web search system that provides access to the structure and nomenclature authority files used for the identification of chemical substances cited in National Library of Medicine (NLM) databases, including the TOXNET system. | |
Record name | Cytidine, 3'-deoxy-3'-fluoro- | |
Source | EPA DSSTox | |
URL | https://comptox.epa.gov/dashboard/DTXSID50154007 | |
Description | DSSTox provides a high quality public chemistry resource for supporting improved predictive toxicology. | |
Retrosynthesis Analysis
AI-Powered Synthesis Planning: Our tool employs the Template_relevance Pistachio, Template_relevance Bkms_metabolic, Template_relevance Pistachio_ringbreaker, Template_relevance Reaxys, Template_relevance Reaxys_biocatalysis model, leveraging a vast database of chemical reactions to predict feasible synthetic routes.
One-Step Synthesis Focus: Specifically designed for one-step synthesis, it provides concise and direct routes for your target compounds, streamlining the synthesis process.
Accurate Predictions: Utilizing the extensive PISTACHIO, BKMS_METABOLIC, PISTACHIO_RINGBREAKER, REAXYS, REAXYS_BIOCATALYSIS database, our tool offers high-accuracy predictions, reflecting the latest in chemical research and data.
Strategy Settings
Precursor scoring | Relevance Heuristic |
---|---|
Min. plausibility | 0.01 |
Model | Template_relevance |
Template Set | Pistachio/Bkms_metabolic/Pistachio_ringbreaker/Reaxys/Reaxys_biocatalysis |
Top-N result to add to graph | 6 |
Feasible Synthetic Routes
Avertissement et informations sur les produits de recherche in vitro
Veuillez noter que tous les articles et informations sur les produits présentés sur BenchChem sont destinés uniquement à des fins informatives. Les produits disponibles à l'achat sur BenchChem sont spécifiquement conçus pour des études in vitro, qui sont réalisées en dehors des organismes vivants. Les études in vitro, dérivées du terme latin "in verre", impliquent des expériences réalisées dans des environnements de laboratoire contrôlés à l'aide de cellules ou de tissus. Il est important de noter que ces produits ne sont pas classés comme médicaments et n'ont pas reçu l'approbation de la FDA pour la prévention, le traitement ou la guérison de toute condition médicale, affection ou maladie. Nous devons souligner que toute forme d'introduction corporelle de ces produits chez les humains ou les animaux est strictement interdite par la loi. Il est essentiel de respecter ces directives pour assurer la conformité aux normes légales et éthiques en matière de recherche et d'expérimentation.