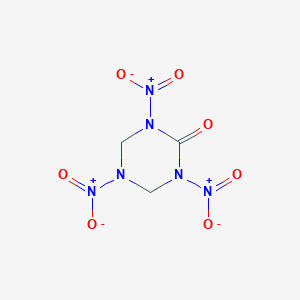
1,3,5-Trinitro-1,3,5-triazinan-2-one
Vue d'ensemble
Description
1,3,5-Trinitro-1,3,5-triazinan-2-one (molecular formula: C₃H₄N₆O₇; molecular weight: 236.100 Da) is a nitrogen-rich heterocyclic compound characterized by a six-membered triazinanone ring substituted with three nitro (-NO₂) groups. The compound’s ChemSpider ID is 9108698, and it is alternatively named 2-oxo-1,3,5-trinitro-1,3,5-triazacyclohexane .
Méthodes De Préparation
Historical Context and Conventional Synthesis
Bachman Process
The Bachman process, developed in the 1940s, remains the most widely used industrial method for synthesizing 1,3,5-trinitro-1,3,5-triazacyclohexane. This two-step procedure involves nitrating hexamethylenetetramine (hexamine) in a mixture of nitric acid and acetic anhydride. The reaction proceeds via the intermediate hexamine nitrate, which undergoes nitrolysis to form the final product .
Key challenges include the formation of the contaminant 1,3,5,7-tetranitro-1,3,5,7-tetrazacyclooctane (HMX), which constitutes up to 10% of the crude product . Subsequent purification steps, such as recrystallization from acetone or nitric acid, reduce HMX levels but lower the overall yield to approximately 60–70% .
Direct Nitration with Ammonium Salts
Modifications to the Bachman method involve adding ammonium nitrate or sulfate to concentrated nitric acid. These salts act as stabilizers, mitigating exothermic decomposition and improving reaction control . While this approach reduces byproduct formation, the requirement for large volumes of acetic anhydride raises costs and safety concerns due to its corrosive and explosive nature .
Modern Innovations in Synthesis
Dinitrogen Pentoxide (N₂O₅) Nitration
A breakthrough method described in USH447H utilizes dinitrogen pentoxide dissolved in 100% nitric acid under a chlorinated hydrocarbon phase (e.g., CCl₄) . This single-step nitrolysis of hexamine at -20°C achieves a 57% yield of 1,3,5-trinitro-1,3,5-triazacyclohexane with no detectable HMX contamination .
Reaction Conditions:
-
Nitrating Agent: 20–30% N₂O₅ in HNO₃
-
Temperature: -20°C
-
Solvent System: CCl₄ (organic phase) and HNO₃ (aqueous phase)
-
Workup: Extraction with ethyl acetate, neutralization with NaHCO₃, and vacuum drying .
This method eliminates acetic anhydride, reducing costs and simplifying waste management. The stability of N₂O₅ in HNO₃ at low temperatures enables scalable production without intermediate purification .
Comparative Analysis of Methods
Parameter | Bachman Process | N₂O₅ Nitration | Copper-Catalyzed |
---|---|---|---|
Yield | 60–70% | 57% | Not Reported |
Purity | 90–95% | >99% | >95% |
Byproducts | HMX (5–10%) | None | Minimal |
Reaction Time | 4–6 hours | 30 minutes | 12–24 hours |
Scalability | Industrial | Pilot-Scale | Lab-Scale |
Environmental Impact | High (Ac₂O waste) | Moderate | Low |
Mechanistic Insights
Nitrolysis Pathways
The nitrolysis of hexamine involves sequential substitution of amine hydrogens with nitro groups. In the Bachman process, acetic anhydride acts as a dehydrating agent, facilitating the formation of the nitronium ion (NO₂⁺) . By contrast, N₂O₅ in HNO₃ directly provides NO₂⁺, bypassing the need for acetic anhydride and reducing side reactions .
Role of Solvent Systems
The biphasic system in N₂O₅ nitration (CCl₄/HNO₃) enhances product isolation by partitioning the hydrophobic nitramine into the organic phase. This prevents over-nitration and stabilizes the reactive intermediates .
Analyse Des Réactions Chimiques
Types of Reactions
1,3,5-Trinitro-1,3,5-triazinan-2-one undergoes several types of chemical reactions, including:
Oxidation: The compound can be oxidized to form various nitrogen oxides and carbon dioxide.
Substitution: The nitro groups can be substituted with other functional groups under specific conditions.
Common Reagents and Conditions
Oxidation: Common oxidizing agents include potassium permanganate and hydrogen peroxide.
Reduction: Reducing agents such as hydrogen gas in the presence of a catalyst or metal hydrides are used.
Substitution: Substitution reactions often require the presence of a base or acid catalyst.
Major Products Formed
Applications De Recherche Scientifique
Military Applications
RDX is primarily utilized in military explosives due to its high stability and energy output. It serves as a key ingredient in various munitions and plastic explosives like C-4. Its effectiveness as an explosive agent makes it a preferred choice for both demolition and military operations .
Environmental Remediation
RDX contamination is a significant environmental concern, particularly at military sites where it has been extensively used. Research has focused on bioremediation methods to degrade RDX in contaminated soils and groundwater. Various microbial strains have been identified that can metabolize RDX into less harmful substances:
- Klebsiella pneumoniae : This bacterium has shown efficacy in degrading RDX to formaldehyde and methanol through anaerobic processes .
- Rhodococcus sp. : This strain catalyzes the biotransformation of RDX via cytochrome P450 enzymes, leading to double denitration .
Biodegradation Studies
Biodegradation studies are crucial for understanding how RDX can be effectively removed from the environment. Key findings include:
- Pathways of Degradation : RDX undergoes sequential reduction to various metabolites such as hexahydro-1-nitroso-3,5-dinitro-1,3,5-triazine (MNX) and hexahydro-1,3-dinitroso-5-nitro-1,3,5-triazine (DNX) before being fully mineralized into carbon dioxide and nitrogen gas .
Table 1: Biodegradation Pathways of RDX
Compound | Degradation Products | Microbial Strain | Reference |
---|---|---|---|
RDX | Formaldehyde, Methanol | Klebsiella pneumoniae SCZ-1 | |
MNX | Formaldehyde, Nitrous Oxide | Klebsiella pneumoniae SCZ-1 | |
RDX | Nitrite, Ammonium | Rhodococcus sp. |
Toxicological Studies
Toxicological assessments have been conducted to evaluate the health risks associated with RDX exposure. The U.S. Environmental Protection Agency (EPA) has compiled extensive data on the toxicological effects of RDX on various organ systems. These studies indicate potential neurotoxic effects and carcinogenic risks from prolonged exposure .
Analytical Techniques for Detection
Various analytical methods have been developed for detecting RDX in environmental samples:
- Chromatography : Liquid and gas chromatography are standard techniques used for quantifying RDX concentrations in soil and water samples.
- Biosensors : Emerging technologies include biosensors that provide rapid on-site detection capabilities for RDX contamination .
Experimental Studies on Vapor Pressure
Recent experimental studies have focused on determining the vapor pressures of RDX under varying temperatures. Understanding these properties is essential for assessing its behavior in different environmental conditions and improving safety protocols during handling .
Mécanisme D'action
The mechanism of action of 1,3,5-Trinitro-1,3,5-triazinan-2-one involves the rapid decomposition of the compound upon initiation. The nitro groups undergo homolytic cleavage, leading to the formation of reactive nitrogen and oxygen species . These reactive species further react with the surrounding molecules, resulting in the release of a large amount of energy in the form of heat and gas . The molecular targets include the nitro groups and the triazinane ring, which undergoes ring cleavage during the decomposition process .
Comparaison Avec Des Composés Similaires
Comparison with Structurally Related Compounds
4,6-Bis(nitroimino)-1,3,5-triazinan-2-one (DNAM)
- Structure: DNAM contains two nitroimino (-NH-NO₂) groups at positions 4 and 6, contrasting with the three nitro groups in 1,3,5-Trinitro-1,3,5-triazinan-2-one.
- Properties: DNAM is classified as an insensitive munition compound due to its lower sensitivity to shock and friction compared to conventional explosives like RDX . Computational studies (B3LYP, M06-2X, MP2) reveal that DNAM exists in multiple tautomeric forms, influencing its stability and reactivity. The nitroimino groups enhance hydrogen bonding, contributing to its insensitivity .
Property | This compound | DNAM |
---|---|---|
Molecular Formula | C₃H₄N₆O₇ | C₃H₄N₈O₅ |
Key Substituents | 3 × -NO₂ | 2 × -NH-NO₂ |
Sensitivity | Likely high (untested) | Low (insensitive munition) |
Tautomerism | Not observed | Multiple tautomeric forms |
Applications | Explosives (theoretical) | Insensitive munitions |
Hexahydro-1,3,5-trinitro-1,3,5-triazine (RDX)
- Structure: RDX is a triazine (six-membered ring with three N atoms) with three nitro groups, lacking the oxygenated ketone group present in triazinanones.
- Properties :
- Explosive Performance : RDX has a detonation velocity of 8,750 m/s, making it a benchmark high explosive. Its mechanical properties in polymer-bonded explosives (PBXs) are influenced by crystal morphology; the (020) face interacts favorably with HTPB-DOA binders, enhancing ductility .
- Environmental Impact : RDX is biodegradable under anaerobic conditions via pathways involving acetylated intermediates, but its nitro groups resist hydrolysis .
Substituted Triazinanones (5-Methyl, 5-Cyclohexyl)
- 5-Methyl-1,3,5-triazinan-2-one: A non-explosive analog with a methyl group at position 4. It has a lower molecular weight (115.13 Da) and higher boiling point (328°C) due to reduced polarity .
- 1,3,5-Tricyclohexyl-1,3,5-triazinan-2-one :
Property | This compound | 5-Methyl Derivative | 5-Cyclohexyl Derivative |
---|---|---|---|
Substituents | 3 × -NO₂ | 1 × -CH₃ | 3 × Cyclohexyl |
Boiling Point | Not reported | 328°C | >300°C (estimated) |
Applications | Energetic materials | Chemical synthesis | Pharmaceutical intermediates |
Key Findings and Implications
- Structural Influence on Sensitivity: The substitution of nitroimino groups (DNAM) or bulky alkyl chains (cyclohexyl) reduces sensitivity compared to nitro-substituted triazinanones, aligning with the trend observed in RDX derivatives .
- Environmental Persistence : Nitro groups in this compound may hinder biodegradation, similar to RDX, necessitating remediation strategies like Fenton oxidation or zero-valent iron reduction .
Activité Biologique
1,3,5-Trinitro-1,3,5-triazinan-2-one (commonly known as RDX or hexogen) is a high-energy compound widely used in military and industrial applications. Its biological activity has been the subject of extensive research due to its potential health impacts and environmental effects. This article provides a detailed overview of the biological activity of RDX, including its mechanisms of action, toxicity profiles, and relevant case studies.
RDX is a cyclic nitramine with the molecular formula and a molecular weight of approximately 222.13 g/mol. It is characterized by its high stability and explosive properties under specific conditions.
Mechanisms of Biological Activity
RDX's biological activity primarily stems from its interaction with biological systems at the molecular level. The compound can undergo metabolic transformations that lead to the formation of reactive intermediates capable of causing cellular damage.
Key Mechanisms:
- Reactive Oxygen Species (ROS) Generation: RDX metabolism can produce ROS, leading to oxidative stress and subsequent cellular damage.
- Enzyme Inhibition: RDX and its metabolites may inhibit various enzymes, affecting metabolic pathways and cellular functions.
- DNA Interaction: There is evidence suggesting that RDX can interact with DNA, potentially leading to mutagenic effects.
Toxicity Profiles
The toxicity of RDX has been extensively documented through various studies involving animal models and human exposure cases.
Case Studies
-
Animal Studies:
- A study involving monkeys exposed to RDX showed symptoms such as hyperirritability and convulsions at higher doses (10 mg/kg). Autopsies revealed congestion in lungs and gastrointestinal tracts .
- In a chronic toxicity study on rats, significant mortality was observed in high-dose groups (50 mg/kg), with symptoms including weight loss and neurological effects like convulsions .
- Human Exposure:
Biological Effects
RDX exposure has been linked to several adverse health effects:
- Neurological Effects: Symptoms such as tremors, convulsions, and behavioral changes have been reported in both animal models and humans exposed to high concentrations of RDX.
- Hematological Changes: Chronic exposure has been associated with alterations in blood parameters, including reduced hemoglobin levels and changes in red blood cell counts .
- Reproductive Toxicity: Studies indicate potential reproductive effects, although more research is needed to fully understand these implications.
Data Tables
Study Type | Subject | Dose (mg/kg) | Observed Effects |
---|---|---|---|
Animal Study | Monkeys | 0.1 - 10 | Hyperirritability, convulsions |
Chronic Exposure | Rats | 15 - 50 | Mortality, weight loss |
Human Case Report | Factory Workers | Variable | Nervous system disturbances |
Q & A
Q. Basic: What are the recommended methods for synthesizing 1,3,5-Trinitro-1,3,5-triazinan-2-one with high purity?
Answer:
The synthesis typically involves nitration of a triazinanone precursor under controlled conditions. A modified Bachmann process (used for RDX synthesis) can be adapted by substituting hexamine with 1,3,5-triazinan-2-one derivatives. Key steps include:
- Acetylation : Pre-functionalization of the triazinanone core to stabilize intermediates.
- Nitration : Use of mixed acid (HNO₃/H₂SO₄) at low temperatures (0–5°C) to minimize side reactions.
- Purification : Recrystallization from acetone/water mixtures to achieve >98% purity, verified via HPLC and elemental analysis (C: 15.2%, N: 35.6%, O: 29.7%) .
Q. Advanced: How can researchers resolve discrepancies in thermal stability data during decomposition studies?
Answer:
Discrepancies often arise from differences in sample preparation (e.g., crystallinity, impurities) or experimental setups. To address this:
- Controlled Conditions : Use standardized DSC/TGA protocols (heating rate: 10°C/min, N₂ atmosphere) to compare decomposition onset temperatures (reported range: 180–220°C).
- Kinetic Analysis : Apply the Kissinger method to calculate activation energies (Eₐ ≈ 150–180 kJ/mol) and identify autocatalytic pathways.
- In Situ Spectroscopy : Pair thermal data with FTIR or Raman to detect intermediate species (e.g., NO₂ radicals) that influence stability .
Q. Basic: What spectroscopic techniques are optimal for structural elucidation of this compound?
Answer:
- X-ray Crystallography : Resolves chair conformations of the triazinanone ring and nitro group orientations (bond angles: C-N-O₂ ~120°) .
- NMR : ¹³C NMR shows distinct carbonyl (C=O) resonance at ~160 ppm, while ¹H NMR reveals axial/equatorial proton splitting in the triazinanone ring (δ = 4.2–5.0 ppm).
- IR Spectroscopy : Confirms nitro (1530 cm⁻¹, asymmetric stretch) and carbonyl (1720 cm⁻¹) functional groups .
Q. Advanced: What are the challenges in detecting environmental contamination of this compound in aqueous systems?
Answer:
- Low Solubility : The compound’s limited water solubility (<50 mg/L) necessitates preconcentration via solid-phase extraction (C18 columns).
- Matrix Interference : Co-eluting organics in wastewater require HPLC-MS/MS with MRM transitions (e.g., m/z 236 → 46 for NO₂⁻ fragments).
- Quantification Limits : Achieve detection thresholds of 0.1 ppb using EPA Method 8330B, optimized for nitroaromatics .
Q. Advanced: How do microbial degradation pathways of this compound interact with abiotic factors?
Answer:
- Biotic Pathways : Geobacter spp. reduce nitro groups via nitroreductases, producing aminodinitro intermediates (LC₅₀ ~5 mg/L for aquatic organisms).
- Abiotic Synergy : Photolysis under UV (λ = 254 nm) generates reactive oxygen species (ROS), accelerating ring cleavage.
- Combined Systems : Sequential bioremediation (microbial consortia) followed by ozonation achieves >90% mineralization in soil/water matrices .
Q. Basic: What safety protocols are critical for handling this compound in laboratory settings?
Answer:
- Explosivity Mitigation : Store in inert atmospheres (Ar/N₂) at ≤5°C; avoid friction, sparks, or sudden temperature changes.
- Personal Protective Equipment (PPE) : Use flame-resistant lab coats, nitrile gloves, and face shields during synthesis.
- Emergency Response : For spills, neutralize with 10% sodium bicarbonate and collect residues in approved explosive waste containers .
Q. Advanced: How can computational modeling predict the environmental fate of this compound?
Answer:
- QSAR Models : Estimate log Kow (1.8–2.3) and soil sorption coefficients (Koc = 200–300 mL/g) to assess bioaccumulation risks.
- PBPK Modeling : Simulate pharmacokinetics in murine models (e.g., female B6C3F1 mice) to extrapolate human exposure thresholds.
- DFT Calculations : Predict redox potentials for nitro group reduction, aligning with experimental voltammetry data .
Q. Tables
Q. Table 1: Key Physicochemical Properties
Property | Value | Method/Source |
---|---|---|
Molecular Formula | C₃H₄N₆O₇ | ChemSpider |
Melting Point | 180–185°C (decomposes) | DSC |
Log Kow | 1.8–2.3 | QSAR |
Aqueous Solubility | <50 mg/L | EPA 8330B |
Q. Table 2: Thermal Decomposition Parameters
Parameter | Value | Conditions |
---|---|---|
Activation Energy (Eₐ) | 150–180 kJ/mol | Kissinger Method |
Onset Temperature | 180–220°C | TGA (N₂, 10°C/min) |
Propriétés
IUPAC Name |
1,3,5-trinitro-1,3,5-triazinan-2-one | |
---|---|---|
Source | PubChem | |
URL | https://pubchem.ncbi.nlm.nih.gov | |
Description | Data deposited in or computed by PubChem | |
InChI |
InChI=1S/C3H4N6O7/c10-3-5(8(13)14)1-4(7(11)12)2-6(3)9(15)16/h1-2H2 | |
Source | PubChem | |
URL | https://pubchem.ncbi.nlm.nih.gov | |
Description | Data deposited in or computed by PubChem | |
InChI Key |
UBBZKFUIBVLJCP-UHFFFAOYSA-N | |
Source | PubChem | |
URL | https://pubchem.ncbi.nlm.nih.gov | |
Description | Data deposited in or computed by PubChem | |
Canonical SMILES |
C1N(CN(C(=O)N1[N+](=O)[O-])[N+](=O)[O-])[N+](=O)[O-] | |
Source | PubChem | |
URL | https://pubchem.ncbi.nlm.nih.gov | |
Description | Data deposited in or computed by PubChem | |
Molecular Formula |
C3H4N6O7 | |
Source | PubChem | |
URL | https://pubchem.ncbi.nlm.nih.gov | |
Description | Data deposited in or computed by PubChem | |
DSSTOX Substance ID |
DTXSID00448887 | |
Record name | 1,3,5-Trinitro-1,3,5-triazinan-2-one | |
Source | EPA DSSTox | |
URL | https://comptox.epa.gov/dashboard/DTXSID00448887 | |
Description | DSSTox provides a high quality public chemistry resource for supporting improved predictive toxicology. | |
Molecular Weight |
236.10 g/mol | |
Source | PubChem | |
URL | https://pubchem.ncbi.nlm.nih.gov | |
Description | Data deposited in or computed by PubChem | |
CAS No. |
115029-35-1 | |
Record name | 1,3,5-Trinitro-1,3,5-triazinan-2-one | |
Source | EPA DSSTox | |
URL | https://comptox.epa.gov/dashboard/DTXSID00448887 | |
Description | DSSTox provides a high quality public chemistry resource for supporting improved predictive toxicology. | |
Avertissement et informations sur les produits de recherche in vitro
Veuillez noter que tous les articles et informations sur les produits présentés sur BenchChem sont destinés uniquement à des fins informatives. Les produits disponibles à l'achat sur BenchChem sont spécifiquement conçus pour des études in vitro, qui sont réalisées en dehors des organismes vivants. Les études in vitro, dérivées du terme latin "in verre", impliquent des expériences réalisées dans des environnements de laboratoire contrôlés à l'aide de cellules ou de tissus. Il est important de noter que ces produits ne sont pas classés comme médicaments et n'ont pas reçu l'approbation de la FDA pour la prévention, le traitement ou la guérison de toute condition médicale, affection ou maladie. Nous devons souligner que toute forme d'introduction corporelle de ces produits chez les humains ou les animaux est strictement interdite par la loi. Il est essentiel de respecter ces directives pour assurer la conformité aux normes légales et éthiques en matière de recherche et d'expérimentation.