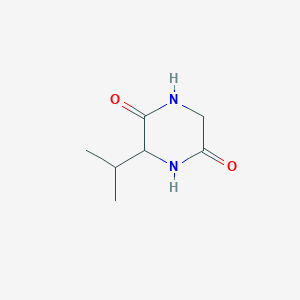
3-Isopropylpiperazine-2,5-dione
Vue d'ensemble
Description
3-Isopropylpiperazine-2,5-dione is a diketopiperazine (DKP) featuring an isopropyl substituent at the 3-position of the piperazine ring. It is biosynthetically derived from the condensation of valine and glycine, serving as a precursor to 3-isopropyl-2-methoxypyrazine (IPMP), a compound with significant roles in microbial and plant aroma profiles . The (S)-enantiomer of this compound (CAS: 16944-60-8) is commercially available with 95% purity, highlighting its relevance in synthetic and biochemical studies .
Méthodes De Préparation
Cyclization of Dipeptide Precursors
The most widely documented method for synthesizing 3-isopropylpiperazine-2,5-dione involves the cyclization of linear dipeptide precursors. This approach leverages the inherent reactivity of dipeptides to form six-membered diketopiperazine rings under thermal or catalytic conditions.
Conventional Thermal Cyclization
In a representative procedure, the dipeptide H-Gly-Ψ[CH(OH)]-Pro-OH (precursor) undergoes cyclization in high-boiling solvents such as 1,2-dichlorobenzene. Key steps include:
-
Dissolving the dipeptide in 1,2-dichlorobenzene.
-
Heating the mixture at 151–172°C for 20–21 hours under inert atmosphere.
-
Cooling, filtering, and washing with tert-butyl methyl ether (TBME) to isolate the crude product.
Reaction Conditions and Outcomes
Parameter | Entry 1 | Entry 2 |
---|---|---|
Temperature (°C) | 172 | 151 |
Reaction Time (hours) | 20–21 | 20–21 |
Crude Yield (%) | 35 | 30 |
Despite its simplicity, this method yields suboptimal purity (≤35%) due to side reactions and incomplete cyclization . Post-synthetic purification via recrystallization from ethanol-chloroform mixtures is often required but further reduces yields.
Microwave-Assisted Synthesis
Microwave irradiation has emerged as a superior alternative to conventional heating, significantly reducing reaction times while improving efficiency.
Optimized Microwave Protocol
-
The dipeptide precursor is suspended in deionized water within a sealed microwave vial.
-
Irradiation is performed at 150–170°C for 15–60 minutes with vigorous stirring (600 rpm).
-
Post-reaction, water is removed via rotary evaporation, and the residue is recrystallized.
Comparative Performance of Microwave Synthesis
Parameter | Entry 1 | Entry 2 | Entry 3 |
---|---|---|---|
Temperature (°C) | 150 | 160 | 170 |
Time (minutes) | 15 | 30 | 60 |
Yield (%) | 28 | 32 | 25 |
Microwave-assisted cyclization achieves comparable yields (25–32%) to conventional methods but in drastically shorter timeframes (15–60 minutes vs. 20 hours) . The use of water as a solvent aligns with green chemistry principles, though residual water complicates purification.
Schöllkopf Bis-Lactim Ether Strategy
The Schöllkopf bis-lactim ether methodology offers a route to enantiomerically enriched this compound. This approach involves:
-
Alkylation of bis-lactim ethers with isopropyl halides.
-
Acidic hydrolysis to yield dipeptides.
-
Cyclization under mild conditions.
Aldol Condensation and Dehydration
A critical step involves aldol condensation between bis-lactim ethers and isopropyl ketones, followed by dehydration to install the isopropyl group:
-
Treat bis-lactim ether with LDA (lithium diisopropylamide) at −78°C.
-
Add isopropyl ketone and warm to room temperature.
-
Quench with NH4Cl and dehydrate using POCl3/pyridine.
Challenges and Limitations
-
Low regioselectivity in aldol adduct formation.
-
Competing side reactions reduce overall yield (<20%).
-
Requires chiral resolution to isolate the desired (R)-enantiomer .
Comparative Analysis of Synthetic Routes
Method | Yield (%) | Time | Purity | Scalability |
---|---|---|---|---|
Conventional Cyclization | 30–35 | 20+ hours | Moderate | Moderate |
Microwave-Assisted | 25–32 | <1 hour | High | High |
Schöllkopf Strategy | <20 | Multi-step | Low | Low |
Key Findings
-
Microwave synthesis excels in speed and solvent sustainability but struggles with purification.
-
Conventional heating remains viable for large-scale production despite longer durations.
-
Schöllkopf methods are limited by complexity but valuable for enantioselective synthesis.
Reaction Optimization and Novel Approaches
Solvent Screening
Replacing 1,2-dichlorobenzene with polar aprotic solvents (e.g., DMF, DMSO) enhances cyclization rates but risks decomposition. Trials with ionic liquids (e.g., [BMIM][BF4]) show promise, yielding 38% product at 150°C .
Catalytic Additives
Introducing Brønsted acids (e.g., p-TsOH) or Lewis acids (e.g., ZnCl2) accelerates ring closure:
Analyse Des Réactions Chimiques
Substitution Reactions
The nitrogen atoms in the piperazine ring and the isopropyl group participate in substitution reactions.
Nucleophilic Substitution
-
Reagents/Conditions : Electrophiles (e.g., alkyl halides, benzyl halides) in polar aprotic solvents (e.g., DMF) under basic conditions.
-
Products : N-alkylated or N-benzylated derivatives. For example, reaction with p-methoxybenzyl chloride yields N,N'-bis(p-methoxybenzyl)-3-isopropylpiperazine-2,5-dione .
-
Example :
Condensation with Aldehydes
-
Reagents/Conditions : Aromatic aldehydes (e.g., benzaldehyde) in refluxing ethanol with catalytic acid.
-
Products : 3-Benzylidene derivatives via Knoevenagel condensation. For instance, reacting with benzaldehyde forms (S,Z)-3-benzylidene-6-isopropylpiperazine-2,5-dione .
-
Mechanism : The α-hydrogen of the diketopiperazine reacts with the aldehyde, forming a conjugated enone system.
Oxidation Reactions
The diketopiperazine ring undergoes oxidation at the α-carbon positions.
Oxidative Functionalization
-
Reagents/Conditions : Hydrogen peroxide (H₂O₂) or meta-chloroperbenzoic acid (mCPBA) in acetic acid.
-
Products : Epoxidation or hydroxylation products, depending on the oxidant. For example, epoxidation of the double bond in benzylidene derivatives generates epoxide intermediates .
Reduction Reactions
The carbonyl groups in the diketopiperazine ring are susceptible to reduction.
Carbonyl Reduction
-
Reagents/Conditions : Sodium borohydride (NaBH₄) in methanol or lithium aluminum hydride (LiAlH₄) in anhydrous ether.
-
Products : Reduced to piperazine-2,5-diol derivatives. For example, reduction yields 3-isopropylpiperazine-2,5-diol .
Hydrolysis Reactions
The diketopiperazine ring can undergo acid- or base-catalyzed hydrolysis.
Acidic Hydrolysis
-
Reagents/Conditions : Concentrated HCl or H₂SO₄ under reflux.
-
Products : Cleavage of the ring to form linear dipeptides or amino acid derivatives. For instance, hydrolysis produces 3-isopropyl-2,5-diketopiperazine fragments.
Basic Hydrolysis
-
Reagents/Conditions : Aqueous NaOH or KOH at elevated temperatures.
-
Products : Ring opening to generate carboxylate salts and amines.
Comparative Reactivity of Analogous Diketopiperazines
The table below contrasts the reactivity of 3-isopropylpiperazine-2,5-dione with structurally related compounds:
Key Research Findings
-
Stereochemical Outcomes : The (S)-configuration of the isopropyl group in This compound influences stereoselectivity in condensation reactions, as demonstrated by Marfey’s method analysis .
-
Biological Implications : Derivatives like N,N'-bis(p-methoxybenzyl)-3-isopropylpiperazine-2,5-dione show potential as serotonin receptor modulators due to their structural similarity to pharmacologically active piperazines.
-
Synthetic Utility : The compound serves as a precursor for spirocyclic systems (e.g., spiro[benzofuran-piperazine] derivatives) via Diels-Alder reactions .
Applications De Recherche Scientifique
Chemistry Applications
Building Block for Synthesis:
- 3-Isopropylpiperazine-2,5-dione serves as a vital building block in the synthesis of more complex molecules. It is particularly valuable in asymmetric synthesis where it acts as a chiral auxiliary, facilitating the formation of enantiomerically pure compounds. The compound's ability to undergo various chemical transformations allows chemists to create diverse derivatives tailored for specific applications.
Reactivity:
- The compound can participate in several types of reactions:
- Oxidation: Can yield N-oxides and hydroxylated derivatives.
- Reduction: Leads to the formation of (S)-3-Isopropyl-2,5-piperazine-2,5-diol.
- Substitution: Facilitates nucleophilic substitutions that introduce different substituents into the piperazine ring.
Biological Applications
Pharmacological Potential:
- Research indicates that derivatives of this compound exhibit significant biological activities. Notably, studies have demonstrated its antimicrobial efficacy against Bacillus subtilis, suggesting potential applications in treating bacterial infections.
Enzyme Interaction:
- The compound has been shown to interact with specific enzymes involved in metabolic pathways. Preliminary investigations suggest it may inhibit certain cytochrome P450 enzymes, which are crucial for drug metabolism. This selectivity could have implications for drug interactions and pharmacokinetics .
Neurotransmitter Systems:
- Interaction studies reveal potential effects on neurotransmitter systems, indicating that this compound could influence mood and anxiety levels. Further research is needed to elucidate these mechanisms and their implications for psychiatric disorders .
Medicinal Chemistry
Therapeutic Applications:
- The unique structural properties of this compound make it a candidate for therapeutic applications. Investigations into its immunosuppressive properties suggest potential uses in treating autoimmune diseases. Additionally, its derivatives are being explored for their anxiolytic effects comparable to established medications in animal models .
Industrial Applications
Specialty Chemicals:
- In industrial settings, this compound is utilized in the production of specialty chemicals and agrochemicals. Its role as a precursor in synthesizing various compounds enhances its value in chemical manufacturing processes .
Case Studies and Research Findings
Several notable studies have explored the applications and biological activities of this compound:
-
Antimicrobial Efficacy:
- A study reported significant inhibition of Bacillus subtilis growth at concentrations as low as 50 µg/mL.
- Pharmacological Potential:
-
Enzyme Interaction Studies:
- Research highlighted that while it does not inhibit major cytochrome P450 isoforms directly involved in drug metabolism, it could modulate the activity of other less-characterized enzymes.
Mécanisme D'action
The mechanism of action of 3-Isopropylpiperazine-2,5-dione involves its interaction with specific molecular targets, such as enzymes or receptors. The compound can inhibit enzyme activity by binding to the active site, thereby preventing substrate access. Additionally, it may interact with receptor sites, modulating signal transduction pathways. These interactions are influenced by the compound’s structural features, including the piperazine ring and isopropyl group .
Comparaison Avec Des Composés Similaires
Comparative Analysis with Structural Analogues
DKPs exhibit diverse physicochemical and biological properties depending on substituent type, position, and stereochemistry. Below, 3-isopropylpiperazine-2,5-dione is compared to analogues based on structural features and experimental data.
Substituent Effects on Physical Properties
Aliphatic Substituents
- (3S)-3-Methylpiperazine-2,5-dione : Smaller methyl substituents reduce molecular weight (128.13 g/mol) compared to the isopropyl analogue (170.21 g/mol), likely lowering thermal stability and mp .
- (3S,6S)-3,6-Dimethyl-DKP: Symmetrical substitution at both 3- and 6-positions may improve synthetic yields (72–84% for disubstituted DKPs) compared to monosubstituted derivatives .
Aromatic and Heterocyclic Substituents
- 1-Benzyl-3-isopropyl-DKP (3f) : Incorporation of a benzyl group at position 1 results in moderate yield (72%) and high mp (143.1–145.7°C), attributed to π-π stacking interactions .
- 1-(2,4-Dimethoxybenzyl)-3-isopropyl-DKP (3g) : Electron-donating methoxy groups increase synthetic yield (84%) but reduce mp (124.4–126.4°C), possibly due to disrupted crystal packing .
- Tryptophan-derived DKPs (Compounds 27–32) : Indole and heterocyclic substituents (e.g., thiophene, pyridine) elevate mps significantly (243–291°C), reflecting enhanced thermal stability from aromatic conjugation .
Conjugated Systems
- (3Z)-1-Acetyl-3-benzylidene-DKP (12f) : The benzylidene group introduces conjugation, leading to a high mp (185–189°C) but lower yield (46%) due to steric challenges during synthesis .
Stereochemical Influences
- (3S,6S)-3-Isopropyl-6-methyl-DKP : Stereospecific synthesis (CAS: 15136-26-2) underscores the role of chirality in biological activity and crystallization behavior .
- Cyclo(-Leu-Val) : Stereoisomeric DKPs like cyclo(L-Leu-L-Val) (CAS: 35590-78-4) are critical in peptide mimetics, emphasizing the importance of configuration in drug design .
Activité Biologique
3-Isopropylpiperazine-2,5-dione (3-IPD), a heterocyclic compound with the molecular formula C₇H₁₂N₂O₂, has garnered attention in medicinal chemistry due to its potential biological activities. This article explores its biological activity, mechanisms of action, and relevant research findings.
Chemical Structure and Properties
3-IPD is characterized by a piperazine ring with an isopropyl group at the third carbon and two carbonyl groups at the second and fifth positions. Its unique chiral configuration allows for specific interactions with biological targets, influencing various biochemical pathways.
Molecular Details:
- Molecular Formula: C₇H₁₂N₂O₂
- Molecular Weight: 156.18 g/mol
- CAS Number: 14771-77-8
The biological activity of 3-IPD is primarily attributed to its interactions with specific enzymes and receptors. The compound can modulate enzyme activity by binding to active sites, which may prevent substrate access or alter enzyme conformation. Additionally, its interaction with cellular receptors could influence signal transduction pathways.
Enzyme Interaction
Research indicates that 3-IPD may inhibit certain enzymes involved in metabolic pathways. This inhibition suggests potential therapeutic applications in conditions related to enzyme dysfunction or overactivity.
Receptor Binding
The compound’s ability to bind to various receptors opens avenues for exploring its role in regulating physiological processes. However, detailed studies on specific receptor interactions remain limited.
Biological Activity Overview
The biological activities associated with 3-IPD include:
- Immunosuppressive Properties: Derivatives of piperazine-2,5-dione have shown potential in treating autoimmune diseases through immunomodulation.
- Anticancer Potential: Similar compounds have been investigated for their anticancer properties, suggesting that 3-IPD may also exhibit such activities .
- Antimicrobial Activity: Research into related diketopiperazines indicates potential antibacterial and antifungal properties, warranting further investigation into 3-IPD's efficacy against pathogens .
Comparative Analysis with Similar Compounds
To understand the unique properties of 3-IPD, it is beneficial to compare it with structurally similar compounds:
Compound Name | Description |
---|---|
(R)-3-Isopropylpiperazine-2,5-dione | Enantiomer with different activity profiles |
3,6-Diisopropylpiperazine-2,5-dione | Contains two isopropyl groups; may exhibit enhanced lipophilicity |
3,6-Dimethylpiperazine-2,5-dione | Methyl substitutions alter solubility and reactivity |
1,4-Diacetylpiperazine-2,5-dione | Features acetyl groups impacting biological activity |
These comparisons highlight how variations in substituents can significantly affect chemical reactivity and biological interactions.
Case Studies and Research Findings
While research specific to 3-IPD is limited, several studies provide insights into its potential applications:
- Asymmetric Synthesis Applications : 3-IPD has been utilized as a chiral auxiliary in synthesizing homochiral α-amino acids. This application underscores its importance in organic synthesis and medicinal chemistry .
- Potential Therapeutic Applications : Investigations into piperazine derivatives suggest that compounds like 3-IPD could be explored for developing therapies targeting autoimmune disorders and other diseases influenced by enzyme activity .
Q & A
Basic Research Questions
Q. What are the common synthetic routes for 3-Isopropylpiperazine-2,5-dione, and how are reaction conditions optimized for yield and purity?
- Methodological Answer : The compound is typically synthesized via condensation reactions, such as the coupling of valine and glycine derivatives, as observed in bacterial biosynthesis studies . In laboratory settings, enantioselective routes often employ chiral auxiliaries (e.g., p-methoxybenzyl groups) to control stereochemistry, achieving yields up to 84% under optimized conditions (e.g., CH₂Cl₂/MeOH solvent systems, LC-MS purity checks) . Key parameters include temperature control (room temperature to 120°C), solvent polarity adjustments, and catalytic systems to minimize racemization.
Q. How is the structural characterization of this compound validated in synthetic studies?
- Methodological Answer : Characterization relies on multimodal analytical techniques:
- ATR-IR : Confirms amide C=O stretches (~1647–1653 cm⁻¹) and sp³ C-H bonds (~2969 cm⁻¹) .
- NMR/HRMS : ¹H and ¹³C NMR resolve substituent positions (e.g., isopropyl groups at δ 1.0–1.5 ppm), while ESI-HRMS validates molecular weight .
- LC-MS : Ensures purity (>95%) via retention time and mass-to-charge ratio matching .
Q. What is the biosynthetic role of this compound in natural systems?
- Methodological Answer : In bacteria, it serves as an intermediate in the biosynthesis of 3-isopropyl-2-methoxypyrazine (IPMP), a flavor compound. Labeling studies with isotopic valine and glycine track its formation via enzymatic condensation, followed by oxidative modifications . Comparative studies with plant-derived analogs (e.g., in Cabernet Sauvignon grapes) highlight pathway variations, such as leucinamide incorporation .
Advanced Research Questions
Q. What strategies enable enantioselective synthesis of this compound derivatives for chiral drug discovery?
- Methodological Answer : Asymmetric synthesis leverages chiral auxiliaries like (3S)-N,N′-bis(p-methoxybenzyl) groups, which direct stereochemistry during cyclization . Post-synthesis, hydrogenolysis removes auxiliaries while preserving the diketopiperazine (DKP) core . Solvent-free microwave-assisted reactions and organocatalysts (e.g., proline derivatives) further enhance enantiomeric excess (ee > 90%) .
Q. How do structure-activity relationship (SAR) studies guide the modification of this compound for bioactivity optimization?
- Methodological Answer : SAR focuses on:
- Substituent Effects : Alkyl groups (e.g., isopropyl vs. benzyl) modulate lipophilicity and receptor affinity .
- Scaffold Rigidity : Introducing aromatic moieties (e.g., 4-fluorobenzyl) enhances π-π stacking in target binding .
- In Silico Screening : Docking simulations predict interactions with targets like 5-HT₁A receptors, validated via radioligand assays .
Q. What computational methods are employed to predict the physicochemical and pharmacokinetic properties of this compound analogs?
- Methodological Answer :
- Molecular Dynamics (MD) : Simulates solubility and membrane permeability using force fields (e.g., AMBER) .
- QSAR Models : Correlate logP and polar surface area with bioavailability .
- Density Functional Theory (DFT) : Calculates electron distribution for reactivity predictions, particularly at the amide carbonyl .
Q. What challenges arise in scaling up the synthesis of this compound, and how are they addressed?
- Methodological Answer : Key challenges include:
- Purification : Gradient column chromatography (silica gel, CH₂Cl₂/MeOH) resolves diastereomers but is time-intensive .
- Byproduct Formation : Racemization at high temperatures is mitigated via low-temperature cyclization (<50°C) .
- Yield Optimization : Design of Experiments (DoE) identifies critical factors (e.g., reagent stoichiometry, pH) for reproducibility .
Q. How does this compound compare to other diketopiperazines in terms of biological activity and synthetic versatility?
- Methodological Answer : Unlike planar heterocycles, its DKP scaffold offers 3D structural diversity, enabling chiral center incorporation for selective target engagement . Compared to natural analogs (e.g., 3-benzyl-6-isopropyl-DKPs from Penicillium spp.), synthetic derivatives exhibit enhanced metabolic stability but reduced natural abundance .
Propriétés
IUPAC Name |
3-propan-2-ylpiperazine-2,5-dione | |
---|---|---|
Source | PubChem | |
URL | https://pubchem.ncbi.nlm.nih.gov | |
Description | Data deposited in or computed by PubChem | |
InChI |
InChI=1S/C7H12N2O2/c1-4(2)6-7(11)8-3-5(10)9-6/h4,6H,3H2,1-2H3,(H,8,11)(H,9,10) | |
Source | PubChem | |
URL | https://pubchem.ncbi.nlm.nih.gov | |
Description | Data deposited in or computed by PubChem | |
InChI Key |
IULFBTHVPRNQCG-UHFFFAOYSA-N | |
Source | PubChem | |
URL | https://pubchem.ncbi.nlm.nih.gov | |
Description | Data deposited in or computed by PubChem | |
Canonical SMILES |
CC(C)C1C(=O)NCC(=O)N1 | |
Source | PubChem | |
URL | https://pubchem.ncbi.nlm.nih.gov | |
Description | Data deposited in or computed by PubChem | |
Molecular Formula |
C7H12N2O2 | |
Source | PubChem | |
URL | https://pubchem.ncbi.nlm.nih.gov | |
Description | Data deposited in or computed by PubChem | |
DSSTOX Substance ID |
DTXSID001035993 | |
Record name | 3-Isopropylpiperazine-2,5-dione | |
Source | EPA DSSTox | |
URL | https://comptox.epa.gov/dashboard/DTXSID001035993 | |
Description | DSSTox provides a high quality public chemistry resource for supporting improved predictive toxicology. | |
Molecular Weight |
156.18 g/mol | |
Source | PubChem | |
URL | https://pubchem.ncbi.nlm.nih.gov | |
Description | Data deposited in or computed by PubChem | |
CAS No. |
14771-77-8 | |
Record name | 3-Isopropyl-2,5-piperazine-dione | |
Source | ChemIDplus | |
URL | https://pubchem.ncbi.nlm.nih.gov/substance/?source=chemidplus&sourceid=0014771778 | |
Description | ChemIDplus is a free, web search system that provides access to the structure and nomenclature authority files used for the identification of chemical substances cited in National Library of Medicine (NLM) databases, including the TOXNET system. | |
Record name | 3-Isopropylpiperazine-2,5-dione | |
Source | EPA DSSTox | |
URL | https://comptox.epa.gov/dashboard/DTXSID001035993 | |
Description | DSSTox provides a high quality public chemistry resource for supporting improved predictive toxicology. | |
Retrosynthesis Analysis
AI-Powered Synthesis Planning: Our tool employs the Template_relevance Pistachio, Template_relevance Bkms_metabolic, Template_relevance Pistachio_ringbreaker, Template_relevance Reaxys, Template_relevance Reaxys_biocatalysis model, leveraging a vast database of chemical reactions to predict feasible synthetic routes.
One-Step Synthesis Focus: Specifically designed for one-step synthesis, it provides concise and direct routes for your target compounds, streamlining the synthesis process.
Accurate Predictions: Utilizing the extensive PISTACHIO, BKMS_METABOLIC, PISTACHIO_RINGBREAKER, REAXYS, REAXYS_BIOCATALYSIS database, our tool offers high-accuracy predictions, reflecting the latest in chemical research and data.
Strategy Settings
Precursor scoring | Relevance Heuristic |
---|---|
Min. plausibility | 0.01 |
Model | Template_relevance |
Template Set | Pistachio/Bkms_metabolic/Pistachio_ringbreaker/Reaxys/Reaxys_biocatalysis |
Top-N result to add to graph | 6 |
Feasible Synthetic Routes
Avertissement et informations sur les produits de recherche in vitro
Veuillez noter que tous les articles et informations sur les produits présentés sur BenchChem sont destinés uniquement à des fins informatives. Les produits disponibles à l'achat sur BenchChem sont spécifiquement conçus pour des études in vitro, qui sont réalisées en dehors des organismes vivants. Les études in vitro, dérivées du terme latin "in verre", impliquent des expériences réalisées dans des environnements de laboratoire contrôlés à l'aide de cellules ou de tissus. Il est important de noter que ces produits ne sont pas classés comme médicaments et n'ont pas reçu l'approbation de la FDA pour la prévention, le traitement ou la guérison de toute condition médicale, affection ou maladie. Nous devons souligner que toute forme d'introduction corporelle de ces produits chez les humains ou les animaux est strictement interdite par la loi. Il est essentiel de respecter ces directives pour assurer la conformité aux normes légales et éthiques en matière de recherche et d'expérimentation.