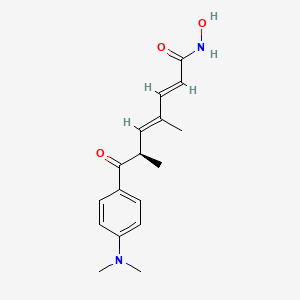
trichostatin A
Vue d'ensemble
Description
Trichostatin A (TSA) is an organic compound that serves as an antifungal antibiotic and selectively inhibits the class I and II mammalian histone deacetylase (HDAC) families of enzymes . It is a potent and specific inhibitor of histone deacetylases (HDACs), which are overexpressed in various cancers and closely correlate with oncogenic factors .
Synthesis Analysis
An efficient synthesis of rac-trichostatic acid and its analogues has been reported starting from a commercially available aldehyde . Further manipulations led to rac-trichostatin A (TSA) .Molecular Structure Analysis
The molecular formula of TSA is C17H22N2O3 . It is a natural derivative of dienohydroxamic acid derived from a fungal metabolite . The molecular weight is 302.37 g/mol .Chemical Reactions Analysis
TSA has been shown to modulate gene expression by interfering with the removal of acetyl groups from histones (histone deacetylases, HDAC) and therefore altering the ability of DNA transcription factors to access the DNA molecules inside chromatin . It also inhibits the HDAC Rpd3, leading to an increase in NHE6, which is a CREB-target gene that regulates cell responses under low-nutrient conditions .Applications De Recherche Scientifique
Trichostatin A (TSA) Applications in Scientific Research
Cancer Therapy: TSA has been studied for its potential in cancer therapy due to its ability to selectively target over-expressed and over-activated receptor tyrosine kinases (RTKs) which are common in various cancer cells .
Neuroprotection: Research suggests that TSA may have neuroprotective roles and could potentially improve pathology related to Alzheimer’s disease, although the mechanisms are still being explored .
Developmental Biology: TSA affects developmental processes as indicated by studies showing morphological changes in microspores during reprogramming, with TSA treatments increasing certain morphologies and symmetric divisions .
Pharmacological Enhancement: Studies have shown that TSA can enhance the cytotoxicity of other pharmacological agents, such as cisplatin, by promoting apoptosis and cell death markers .
Cellular Stress Response: TSA has been observed to promote the expression of GRP78, a stress response protein, and improve its binding ability with BDNF, which is crucial for cellular response to high glucose environments .
Mécanisme D'action
Mode of Action
TSA interferes with the removal of acetyl groups from histones by inhibiting HDACs . This alters the ability of DNA transcription factors to access the DNA molecules inside chromatin . As a result, TSA can be used to alter gene expression .
Biochemical Pathways
By inhibiting HDACs, TSA affects the acetylation status of histones, thereby influencing the chromatin structure and gene expression . This can lead to various downstream effects, including the promotion of apoptosis-related genes, which can slow the progression of cancer .
Pharmacokinetics
After administration, TSA is rapidly absorbed and detectable in plasma within 2 minutes . The maximum concentration (Cmax) of 40 μg/ml and 8 ng/ml occurs within 5 minutes, followed by a rapid exponential decay in plasma TSA concentration with a half-life (t1/2) of 6.3 minutes and 9.6 minutes for high and low doses, respectively .
Result of Action
TSA has potential as an anti-cancer drug . It promotes the expression of apoptosis-related genes, leading to cancerous cells surviving at lower rates, thus slowing the progression of cancer . Other mechanisms may include the activity of HDIs to induce cell differentiation, thus acting to “mature” some of the de-differentiated cells found in tumors .
Safety and Hazards
Propriétés
IUPAC Name |
(2E,4E,6R)-7-[4-(dimethylamino)phenyl]-N-hydroxy-4,6-dimethyl-7-oxohepta-2,4-dienamide | |
---|---|---|
Source | PubChem | |
URL | https://pubchem.ncbi.nlm.nih.gov | |
Description | Data deposited in or computed by PubChem | |
InChI |
InChI=1S/C17H22N2O3/c1-12(5-10-16(20)18-22)11-13(2)17(21)14-6-8-15(9-7-14)19(3)4/h5-11,13,22H,1-4H3,(H,18,20)/b10-5+,12-11+/t13-/m1/s1 | |
Source | PubChem | |
URL | https://pubchem.ncbi.nlm.nih.gov | |
Description | Data deposited in or computed by PubChem | |
InChI Key |
RTKIYFITIVXBLE-QEQCGCAPSA-N | |
Source | PubChem | |
URL | https://pubchem.ncbi.nlm.nih.gov | |
Description | Data deposited in or computed by PubChem | |
Canonical SMILES |
CC(C=C(C)C=CC(=O)NO)C(=O)C1=CC=C(C=C1)N(C)C | |
Source | PubChem | |
URL | https://pubchem.ncbi.nlm.nih.gov | |
Description | Data deposited in or computed by PubChem | |
Isomeric SMILES |
C[C@H](/C=C(\C)/C=C/C(=O)NO)C(=O)C1=CC=C(C=C1)N(C)C | |
Source | PubChem | |
URL | https://pubchem.ncbi.nlm.nih.gov | |
Description | Data deposited in or computed by PubChem | |
Molecular Formula |
C17H22N2O3 | |
Source | PubChem | |
URL | https://pubchem.ncbi.nlm.nih.gov | |
Description | Data deposited in or computed by PubChem | |
DSSTOX Substance ID |
DTXSID6037063 | |
Record name | Trichostatin A | |
Source | EPA DSSTox | |
URL | https://comptox.epa.gov/dashboard/DTXSID6037063 | |
Description | DSSTox provides a high quality public chemistry resource for supporting improved predictive toxicology. | |
Molecular Weight |
302.37 g/mol | |
Source | PubChem | |
URL | https://pubchem.ncbi.nlm.nih.gov | |
Description | Data deposited in or computed by PubChem | |
Product Name |
trichostatin A | |
CAS RN |
58880-19-6 | |
Record name | Trichostatin A | |
Source | CAS Common Chemistry | |
URL | https://commonchemistry.cas.org/detail?cas_rn=58880-19-6 | |
Description | CAS Common Chemistry is an open community resource for accessing chemical information. Nearly 500,000 chemical substances from CAS REGISTRY cover areas of community interest, including common and frequently regulated chemicals, and those relevant to high school and undergraduate chemistry classes. This chemical information, curated by our expert scientists, is provided in alignment with our mission as a division of the American Chemical Society. | |
Explanation | The data from CAS Common Chemistry is provided under a CC-BY-NC 4.0 license, unless otherwise stated. | |
Record name | Trichostatin A | |
Source | ChemIDplus | |
URL | https://pubchem.ncbi.nlm.nih.gov/substance/?source=chemidplus&sourceid=0058880196 | |
Description | ChemIDplus is a free, web search system that provides access to the structure and nomenclature authority files used for the identification of chemical substances cited in National Library of Medicine (NLM) databases, including the TOXNET system. | |
Record name | Trichostatin A | |
Source | DrugBank | |
URL | https://www.drugbank.ca/drugs/DB04297 | |
Description | The DrugBank database is a unique bioinformatics and cheminformatics resource that combines detailed drug (i.e. chemical, pharmacological and pharmaceutical) data with comprehensive drug target (i.e. sequence, structure, and pathway) information. | |
Explanation | Creative Common's Attribution-NonCommercial 4.0 International License (http://creativecommons.org/licenses/by-nc/4.0/legalcode) | |
Record name | Trichostatin A | |
Source | EPA DSSTox | |
URL | https://comptox.epa.gov/dashboard/DTXSID6037063 | |
Description | DSSTox provides a high quality public chemistry resource for supporting improved predictive toxicology. | |
Record name | TRICHOSTATIN A | |
Source | FDA Global Substance Registration System (GSRS) | |
URL | https://gsrs.ncats.nih.gov/ginas/app/beta/substances/3X2S926L3Z | |
Description | The FDA Global Substance Registration System (GSRS) enables the efficient and accurate exchange of information on what substances are in regulated products. Instead of relying on names, which vary across regulatory domains, countries, and regions, the GSRS knowledge base makes it possible for substances to be defined by standardized, scientific descriptions. | |
Explanation | Unless otherwise noted, the contents of the FDA website (www.fda.gov), both text and graphics, are not copyrighted. They are in the public domain and may be republished, reprinted and otherwise used freely by anyone without the need to obtain permission from FDA. Credit to the U.S. Food and Drug Administration as the source is appreciated but not required. | |
Retrosynthesis Analysis
AI-Powered Synthesis Planning: Our tool employs the Template_relevance Pistachio, Template_relevance Bkms_metabolic, Template_relevance Pistachio_ringbreaker, Template_relevance Reaxys, Template_relevance Reaxys_biocatalysis model, leveraging a vast database of chemical reactions to predict feasible synthetic routes.
One-Step Synthesis Focus: Specifically designed for one-step synthesis, it provides concise and direct routes for your target compounds, streamlining the synthesis process.
Accurate Predictions: Utilizing the extensive PISTACHIO, BKMS_METABOLIC, PISTACHIO_RINGBREAKER, REAXYS, REAXYS_BIOCATALYSIS database, our tool offers high-accuracy predictions, reflecting the latest in chemical research and data.
Strategy Settings
Precursor scoring | Relevance Heuristic |
---|---|
Min. plausibility | 0.01 |
Model | Template_relevance |
Template Set | Pistachio/Bkms_metabolic/Pistachio_ringbreaker/Reaxys/Reaxys_biocatalysis |
Top-N result to add to graph | 6 |
Feasible Synthetic Routes
Q & A
Q1: What is the primary molecular target of Trichostatin A (TSA)?
A1: Trichostatin A is a potent and specific inhibitor of histone deacetylases (HDACs) [, , , ].
Q2: How does TSA interact with HDACs?
A2: TSA inhibits HDACs by binding to the enzyme through its hydroxamic acid group. This interaction blocks the enzyme's activity [, ]. The dimethylaniline moiety of TSA is also important for its specific recognition by certain HDACs like TsnB9 [].
Q3: What are the downstream effects of HDAC inhibition by TSA?
A3: HDAC inhibition by TSA leads to increased histone acetylation, altering chromatin structure and influencing gene expression [, , ]. This can result in diverse cellular responses, including:
- Induction of cell cycle arrest: TSA can induce cell cycle arrest at the G1/S [] or G2/M phase [, , , ] in various cancer cell lines. This arrest is often associated with upregulation of cyclin-dependent kinase inhibitors like p21WAF1/CIP1 [, , , , ].
- Induction of apoptosis: TSA has been shown to induce apoptosis in various cancer cell lines [, , , , , , , , , , ]. The mechanisms of apoptosis induction can involve both caspase-dependent pathways (activation of caspase-3, -8, -9) [, , ] and modulation of Bcl-2 family proteins [, ].
- Modulation of specific gene expression: Besides p21WAF1/CIP1, TSA can influence the expression of other genes involved in various cellular processes. For example, TSA can downregulate thymidylate synthase (TS), a key enzyme in nucleotide biosynthesis, leading to increased sensitivity to 5-fluorouracil in cancer cells []. It can also influence the expression of genes involved in inflammation (e.g., CD36, TNF-α, VCAM-1) [], potentially impacting processes like atherosclerosis.
Q4: Does TSA affect the expression of all genes equally?
A4: No, TSA does not affect the expression of all genes equally. Microarray studies comparing TSA with another HDAC inhibitor, CG-1521, showed that these compounds have different gene expression profiles []. This suggests that specific HDAC isoforms might be responsible for regulating distinct sets of genes and that selective HDAC inhibition could lead to more targeted therapeutic effects.
Q5: What is the molecular formula and weight of TSA?
A5: While not explicitly provided in these abstracts, the molecular formula of Trichostatin A is C17H22N2O3, and its molecular weight is 302.37 g/mol. This information can be found in chemical databases and research articles focusing on its structure.
Q6: How does the structure of TSA contribute to its HDAC inhibitory activity?
A7: The hydroxamic acid group of TSA is essential for its interaction with the zinc ion in the active site of HDAC enzymes [, ]. Modifications to this group typically lead to a loss or reduction in activity.
Q7: Have any studies investigated the impact of structural modifications on TSA's activity?
A8: Yes, studies investigating valproic acid (VPA) derivatives, structurally similar to TSA, have explored the relationship between structure, HDAC inhibition, and teratogenicity []. These studies highlight the importance of the carboxylic acid function for HDAC inhibition in VPA analogs. Notably, while VPA amides and hydroxamates can cause neural tube defects, they do not inhibit HDACs []. This suggests that the carboxylic acid group, present in VPA but not its amides or hydroxamates, is crucial for HDAC inhibitory activity in this class of compounds. Further research into modified TSA analogs could provide insights into designing more specific and potent HDAC inhibitors.
Q8: What is known about the stability of TSA?
A9: Trichostatin A is known to be rapidly metabolized in vivo, limiting its therapeutic application when administered intravenously [].
Q9: Are there any strategies to improve the stability and bioavailability of TSA?
A10: Yes, encapsulating TSA in liposomes, specifically Stealth® liposomes (TSA-lipo), has been shown to improve its stability, enhance tumor accumulation, and increase its anticancer activity in vivo []. This suggests that liposomal formulations could be a promising approach to overcome the limitations associated with TSA's rapid metabolism and improve its therapeutic potential.
Q10: How is TSA metabolized?
A10: While the specific metabolic pathways of TSA are not extensively discussed in these abstracts, its rapid metabolism likely involves enzymatic degradation, potentially via pathways similar to other hydroxamic acid-containing compounds.
Q11: What in vitro models have been used to study TSA?
A11: Researchers have employed various in vitro models to investigate the effects of TSA, including:
- Cancer cell lines: Numerous studies utilize human cancer cell lines, including breast cancer (MCF-7, T47-D, MDA-MB-231, SKBr-3) [, , ], prostate cancer (PC3, LNCaP, 267B1/K-ras) [, , ], lung cancer (A549, NCI-H1299) [, , ], gastric cancer (SGC-7901, MGC-803) [, ], ovarian cancer (skov-3) [], anaplastic thyroid carcinoma (DRO) [], bladder cancer [], and tongue squamous cell carcinoma (SCC-6) [], to assess its anti-proliferative, apoptotic, and gene regulatory effects.
- Primary cell cultures: Studies have also used primary cultures of cells, such as rabbit perichondrial grafts for cartilage regeneration studies [], and human intestinal epithelial cells to investigate the modulation of complement component biosynthesis [].
- Immune cells: TSA's effects on immune responses have been explored in models using natural killer (NK) cells [].
Q12: What in vivo models have been used to study TSA?
A12: In vivo studies investigating the therapeutic potential of TSA have primarily focused on:
- Xenograft models: Researchers have utilized xenograft models, where human cancer cells are implanted into immunodeficient mice, to evaluate TSA's antitumor efficacy. These models have been used for breast cancer (MCF-7, MDA-MB-231) [] and prostate cancer [] studies.
- Animal models of disease: TSA's effects on atherosclerosis were investigated in LDL receptor-deficient (Ldlr−/−) mice, a model prone to developing atherosclerosis when fed a high-fat diet []. Additionally, TSA's impact on conjunctival fibrosis after trabeculectomy was assessed in a rat model [].
- Developmental models: TSA's effects on embryonic development and teratogenicity have been investigated in the NMRI-exencephaly mouse model []. Additionally, its impact on cartilage regeneration was evaluated in a rabbit perichondrial graft model [].
Q13: Are there known mechanisms of resistance to TSA?
A13: While the provided abstracts do not specifically focus on resistance mechanisms to TSA, potential mechanisms could involve:
Q14: What is known about the toxicity and safety profile of TSA?
A17: While the provided research highlights the therapeutic potential of TSA, it's important to note that HDAC inhibitors, including TSA, can exhibit off-target effects and toxicity. For example, in a study investigating the effects of VPA derivatives (structurally similar to TSA) on neural tube defects, researchers found that while VPA amides and hydroxamates can cause these defects, they do not inhibit HDACs []. This suggests that these compounds might exert teratogenic effects through mechanisms independent of HDAC inhibition. Additionally, in a study assessing the impact of TSA on atherosclerosis in LDL receptor-deficient mice, researchers observed exacerbated atherosclerosis in TSA-treated mice, indicating potential pro-atherogenic effects [].
Q15: What strategies are being explored to improve the delivery of TSA to specific targets?
A18: Liposomal encapsulation, exemplified by TSA-lipo, represents a promising drug delivery strategy to enhance TSA's stability, bioavailability, and tumor targeting []. This approach could potentially minimize off-target effects and improve therapeutic efficacy. Further research into targeted delivery systems, such as antibody-drug conjugates or nanoparticle-based approaches, could further enhance TSA's specificity and therapeutic index.
Q16: What biomarkers are being investigated to predict the efficacy of TSA or monitor treatment response?
A16: Several potential biomarkers have emerged from the research on TSA:
Avertissement et informations sur les produits de recherche in vitro
Veuillez noter que tous les articles et informations sur les produits présentés sur BenchChem sont destinés uniquement à des fins informatives. Les produits disponibles à l'achat sur BenchChem sont spécifiquement conçus pour des études in vitro, qui sont réalisées en dehors des organismes vivants. Les études in vitro, dérivées du terme latin "in verre", impliquent des expériences réalisées dans des environnements de laboratoire contrôlés à l'aide de cellules ou de tissus. Il est important de noter que ces produits ne sont pas classés comme médicaments et n'ont pas reçu l'approbation de la FDA pour la prévention, le traitement ou la guérison de toute condition médicale, affection ou maladie. Nous devons souligner que toute forme d'introduction corporelle de ces produits chez les humains ou les animaux est strictement interdite par la loi. Il est essentiel de respecter ces directives pour assurer la conformité aux normes légales et éthiques en matière de recherche et d'expérimentation.