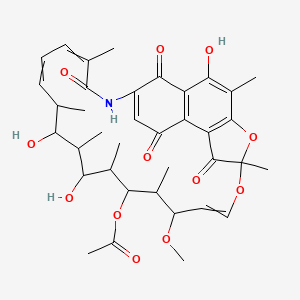
Rifamycin S
Vue d'ensemble
Description
Rifamycin S is an organic molecular entity . It is a natural product found in Amycolatopsis mediterranei and Streptomyces hygroscopicus . It is used to treat traveler’s diarrhea that is caused by a bacteria called Escherichia coli .
Synthesis Analysis
Rifamycin S is synthesized either naturally by the bacterium Amycolatopsis rifamycinica or artificially . The facile synthesis of rifamycin S from rifamycin B, a member of the ansamycin family of antibiotics, via the oxidation of rifamycin B was developed . Currently on an industrial scale, this oxidation is performed using harsh pH conditions and chlorinated solvents .Molecular Structure Analysis
The molecular structure and conformation of rifamycin S is a potent inhibitor of DNA-dependent RNA polymerase . The molecular formula of Rifamycin S is C37H45NO12 .Chemical Reactions Analysis
A network of reactions underlying the biosynthesis of rifamycin SV, S, L, O, and B has been characterized . The two-subunit transketolase Rif15 and the cytochrome P450 enzyme Rif16 are found to mediate, respectively, a unique C–O bond formation in rifamycin L and an atypical P450 ester-to-ether transformation from rifamycin L to BApplications De Recherche Scientifique
Treatment of Tuberculosis
Rifamycin S and its derivatives, such as rifampicin and rifapentine, are commonly used for the treatment of tuberculosis . They exhibit bactericidal activity against many Gram-positive and Gram-negative bacteria by inhibiting RNA polymerase (RNAP) .
Treatment of Mycobacterium Avium Complex Infections
Rifabutin, a derivative of Rifamycin S, is primarily used to treat Mycobacterium avium complex infections .
Treatment of Traveler’s Diarrhea
Rifaximin, another derivative of Rifamycin S, is mostly used to treat traveler’s diarrhea .
Treatment of Staphylococcal Infections
Rifampicin, a derivative of Rifamycin S, is recommended as a component in the treatment of prosthetic joint infections caused by staphylococci .
Treatment of Leprosy and AIDS-related Mycobacterial Infections
Rifamycin S and its derivatives have been used for decades in the clinic for the treatment of leprosy, and AIDS-related mycobacterial infections .
Biosynthesis Research
Rifamycin S plays a crucial role in the biosynthesis of rifamycin SV, S, L, O, and B . The two-subunit transketolase Rif15 and the cytochrome P450 enzyme Rif16 mediate a unique C–O bond formation in rifamycin L and an atypical P450 ester-to-ether transformation from rifamycin L to B .
Enzyme Research
Rifamycin S is used in the study of rifamycin oxidase, an enzyme found in Humicola spp .
Antibiotic Resistance Research
Rifamycin S is used in research on antibiotic resistance. Despite its effectiveness, resistance to rifamycins is prevalent, and the mechanisms range from primary target modification and antibiotic inactivation to cytoplasmic exclusion .
Propriétés
IUPAC Name |
[(7S,9E,11S,12R,13S,14R,15R,16R,17S,18S,19E,21Z)-2,15,17-trihydroxy-11-methoxy-3,7,12,14,16,18,22-heptamethyl-6,23,27,29-tetraoxo-8,30-dioxa-24-azatetracyclo[23.3.1.14,7.05,28]triaconta-1(28),2,4,9,19,21,25-heptaen-13-yl] acetate | |
---|---|---|
Source | PubChem | |
URL | https://pubchem.ncbi.nlm.nih.gov | |
Description | Data deposited in or computed by PubChem | |
InChI |
InChI=1S/C37H45NO12/c1-16-11-10-12-17(2)36(46)38-23-15-24(40)26-27(32(23)44)31(43)21(6)34-28(26)35(45)37(8,50-34)48-14-13-25(47-9)18(3)33(49-22(7)39)20(5)30(42)19(4)29(16)41/h10-16,18-20,25,29-30,33,41-43H,1-9H3,(H,38,46)/b11-10+,14-13+,17-12-/t16-,18+,19+,20+,25-,29-,30+,33+,37-/m0/s1 | |
Source | PubChem | |
URL | https://pubchem.ncbi.nlm.nih.gov | |
Description | Data deposited in or computed by PubChem | |
InChI Key |
BTVYFIMKUHNOBZ-ODRIEIDWSA-N | |
Source | PubChem | |
URL | https://pubchem.ncbi.nlm.nih.gov | |
Description | Data deposited in or computed by PubChem | |
Canonical SMILES |
CC1C=CC=C(C(=O)NC2=CC(=O)C3=C(C2=O)C(=C(C4=C3C(=O)C(O4)(OC=CC(C(C(C(C(C(C1O)C)O)C)OC(=O)C)C)OC)C)C)O)C | |
Source | PubChem | |
URL | https://pubchem.ncbi.nlm.nih.gov | |
Description | Data deposited in or computed by PubChem | |
Isomeric SMILES |
C[C@H]1/C=C/C=C(\C(=O)NC2=CC(=O)C3=C(C2=O)C(=C(C4=C3C(=O)[C@](O4)(O/C=C/[C@@H]([C@H]([C@H]([C@@H]([C@@H]([C@@H]([C@H]1O)C)O)C)OC(=O)C)C)OC)C)C)O)/C | |
Source | PubChem | |
URL | https://pubchem.ncbi.nlm.nih.gov | |
Description | Data deposited in or computed by PubChem | |
Molecular Formula |
C37H45NO12 | |
Source | PubChem | |
URL | https://pubchem.ncbi.nlm.nih.gov | |
Description | Data deposited in or computed by PubChem | |
DSSTOX Substance ID |
DTXSID301043935 | |
Record name | Rifamycin S | |
Source | EPA DSSTox | |
URL | https://comptox.epa.gov/dashboard/DTXSID301043935 | |
Description | DSSTox provides a high quality public chemistry resource for supporting improved predictive toxicology. | |
Molecular Weight |
695.8 g/mol | |
Source | PubChem | |
URL | https://pubchem.ncbi.nlm.nih.gov | |
Description | Data deposited in or computed by PubChem | |
Product Name |
Rifamycin S | |
CAS RN |
13553-79-2 | |
Record name | Rifamycin S | |
Source | CAS Common Chemistry | |
URL | https://commonchemistry.cas.org/detail?cas_rn=13553-79-2 | |
Description | CAS Common Chemistry is an open community resource for accessing chemical information. Nearly 500,000 chemical substances from CAS REGISTRY cover areas of community interest, including common and frequently regulated chemicals, and those relevant to high school and undergraduate chemistry classes. This chemical information, curated by our expert scientists, is provided in alignment with our mission as a division of the American Chemical Society. | |
Explanation | The data from CAS Common Chemistry is provided under a CC-BY-NC 4.0 license, unless otherwise stated. | |
Record name | Rifamycin S | |
Source | ChemIDplus | |
URL | https://pubchem.ncbi.nlm.nih.gov/substance/?source=chemidplus&sourceid=0013553792 | |
Description | ChemIDplus is a free, web search system that provides access to the structure and nomenclature authority files used for the identification of chemical substances cited in National Library of Medicine (NLM) databases, including the TOXNET system. | |
Record name | Rifamycin S | |
Source | EPA DSSTox | |
URL | https://comptox.epa.gov/dashboard/DTXSID301043935 | |
Description | DSSTox provides a high quality public chemistry resource for supporting improved predictive toxicology. | |
Record name | RIFAMYCIN S | |
Source | FDA Global Substance Registration System (GSRS) | |
URL | https://gsrs.ncats.nih.gov/ginas/app/beta/substances/PI53N820JV | |
Description | The FDA Global Substance Registration System (GSRS) enables the efficient and accurate exchange of information on what substances are in regulated products. Instead of relying on names, which vary across regulatory domains, countries, and regions, the GSRS knowledge base makes it possible for substances to be defined by standardized, scientific descriptions. | |
Explanation | Unless otherwise noted, the contents of the FDA website (www.fda.gov), both text and graphics, are not copyrighted. They are in the public domain and may be republished, reprinted and otherwise used freely by anyone without the need to obtain permission from FDA. Credit to the U.S. Food and Drug Administration as the source is appreciated but not required. | |
Retrosynthesis Analysis
AI-Powered Synthesis Planning: Our tool employs the Template_relevance Pistachio, Template_relevance Bkms_metabolic, Template_relevance Pistachio_ringbreaker, Template_relevance Reaxys, Template_relevance Reaxys_biocatalysis model, leveraging a vast database of chemical reactions to predict feasible synthetic routes.
One-Step Synthesis Focus: Specifically designed for one-step synthesis, it provides concise and direct routes for your target compounds, streamlining the synthesis process.
Accurate Predictions: Utilizing the extensive PISTACHIO, BKMS_METABOLIC, PISTACHIO_RINGBREAKER, REAXYS, REAXYS_BIOCATALYSIS database, our tool offers high-accuracy predictions, reflecting the latest in chemical research and data.
Strategy Settings
Precursor scoring | Relevance Heuristic |
---|---|
Min. plausibility | 0.01 |
Model | Template_relevance |
Template Set | Pistachio/Bkms_metabolic/Pistachio_ringbreaker/Reaxys/Reaxys_biocatalysis |
Top-N result to add to graph | 6 |
Feasible Synthetic Routes
Q & A
Q1: What is the mechanism of action of Rifamycin S and its derivatives?
A1: Rifamycin S and its derivatives exert their antibacterial activity by primarily targeting bacterial DNA-dependent RNA polymerase (DDRP) []. This interaction disrupts the initiation step of RNA synthesis, effectively halting bacterial gene expression and leading to cell death [].
Q2: How does Rifamycin S interact with bacterial DDRP at a molecular level?
A2: The interaction involves the formation of non-covalent bonds between specific oxygenated groups on the Rifamycin S molecule and the enzyme's active site []. Key structural features on Rifamycin S crucial for this interaction include oxygen atoms at positions C(1), C(8), C(21), and C(23) [, ]. These oxygen atoms form hydrogen bonds with amino acid residues in the DDRP active site, locking the antibiotic in place and preventing RNA synthesis [].
Q3: What is the significance of the ansa chain in Rifamycin S and how does its conformation influence activity?
A3: The ansa chain, a unique structural feature of ansamycin antibiotics like Rifamycin S, plays a crucial role in the molecule's activity []. The conformation of the ansa chain, particularly the middle portion, is essential for the proper positioning of the oxygenated groups involved in binding to DDRP []. Modification or alteration of this conformation can significantly impact the compound's inhibitory activity [, ].
Q4: Does modifying the 3-position substituent on Rifamycin S affect its activity, and if so, how?
A4: Yes, the nature of the substituent at the 3-position of Rifamycin S significantly influences its activity []. Studies have shown that the chemical properties of this substituent, such as its electronegativity, can impact the conformation of the ansa chain and consequently, the molecule's ability to bind to DDRP [, ]. For instance, bulky or highly electronegative substituents can hinder the proper positioning of the ansa chain, leading to a decrease in activity [].
Q5: What is the molecular formula and weight of Rifamycin S?
A5: The molecular formula of Rifamycin S is C37H45NO12, and its molecular weight is 695.75 g/mol.
Q6: What spectroscopic techniques are commonly used to characterize Rifamycin S and its derivatives?
A6: Various spectroscopic techniques are employed to characterize Rifamycin S and its derivatives, including:
- Nuclear Magnetic Resonance (NMR) spectroscopy: This technique is used to determine the structure and conformation of the ansa chain and other structural features [, , ].
Q7: Can you elaborate on the use of Rifamycin S in the synthesis of other rifamycin derivatives and provide examples?
A7: Rifamycin S serves as a crucial starting material for synthesizing numerous semisynthetic rifamycin derivatives with improved pharmacological properties []. Examples include:
- Rifampicin: A widely used antibiotic for treating tuberculosis, synthesized by introducing a 3-formylhydrazone group to Rifamycin SV, a derivative of Rifamycin S [, ].
- Rifabutin: Another antitubercular agent, synthesized by reacting Rifamycin S with 2-amino-4-methylpyridine [, ].
- Glycosylrifamycins: A class of rifamycin derivatives synthesized by attaching sugar moieties to Rifamycin S or its derivatives, potentially improving pharmacokinetic properties [].
Q8: How is Rifamycin S produced on an industrial scale?
A8: The industrial production of Rifamycin S typically involves a two-step process:
- Fermentation: The bacterium Amycolatopsis mediterranei (formerly known as Nocardia mediterranei) is fermented to produce Rifamycin B [, ].
- Oxidation: Rifamycin B, a less active precursor, is chemically oxidized to Rifamycin S [, ]. Traditionally, this oxidation involved harsh conditions and chlorinated solvents, but recent research focuses on developing more sustainable methods using milder oxidants and greener solvents like methanol [, ].
Q9: What are the advantages of using biocatalytic methods for the production of Rifamycin S?
A9: Employing biocatalytic approaches, such as using enzymes like rifamycin oxidase, for Rifamycin S production offers several advantages:
- Higher selectivity and yield: Enzymes exhibit high substrate specificity, leading to fewer side products and increased yield compared to chemical methods [, ].
- Milder reaction conditions: Enzymatic reactions generally occur under milder conditions (e.g., lower temperature, neutral pH), reducing energy consumption and minimizing the formation of unwanted byproducts [].
- Environmental friendliness: Enzymes are biodegradable and non-toxic, making them environmentally friendly catalysts compared to some harsh chemical reagents [, ].
Q10: What are some of the challenges associated with using biocatalytic approaches for Rifamycin S production?
A10: While biocatalysis presents a promising avenue for sustainable Rifamycin S production, challenges exist:
- Enzyme cost and stability: Some enzymes can be expensive to produce and may exhibit limited stability under industrial conditions [, ].
- Process optimization: Finding the optimal conditions for enzyme activity and stability, such as pH, temperature, and substrate concentration, can be complex [, ].
- Downstream processing: Separating the desired product from the reaction mixture containing the biocatalyst can be challenging [].
Q11: What are the research implications of studying Rifamycin S biotransformation?
A11: Investigating the biotransformation of Rifamycin S offers valuable insights into:
- Biosynthetic pathways: Understanding how microorganisms produce and modify Rifamycin S and its analogs can provide valuable information for developing novel synthetic strategies and engineering more efficient production strains [].
- Enzyme discovery and engineering: Studying enzymes involved in Rifamycin S biosynthesis and modification can lead to discovering novel biocatalysts for various applications, including synthesizing other valuable compounds [, ].
- Drug discovery and development: Investigating the structure-activity relationships and biotransformation pathways of Rifamycin S can aid in designing new derivatives with improved pharmacological properties, such as enhanced activity, reduced toxicity, and overcoming drug resistance [, ].
Avertissement et informations sur les produits de recherche in vitro
Veuillez noter que tous les articles et informations sur les produits présentés sur BenchChem sont destinés uniquement à des fins informatives. Les produits disponibles à l'achat sur BenchChem sont spécifiquement conçus pour des études in vitro, qui sont réalisées en dehors des organismes vivants. Les études in vitro, dérivées du terme latin "in verre", impliquent des expériences réalisées dans des environnements de laboratoire contrôlés à l'aide de cellules ou de tissus. Il est important de noter que ces produits ne sont pas classés comme médicaments et n'ont pas reçu l'approbation de la FDA pour la prévention, le traitement ou la guérison de toute condition médicale, affection ou maladie. Nous devons souligner que toute forme d'introduction corporelle de ces produits chez les humains ou les animaux est strictement interdite par la loi. Il est essentiel de respecter ces directives pour assurer la conformité aux normes légales et éthiques en matière de recherche et d'expérimentation.