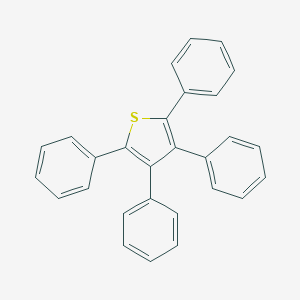
Tetraphenylthiophene
Vue d'ensemble
Description
Tetraphenylthiophene is a chemical compound with the molecular formula C28H20S . It has an average mass of 388.523 Da and a monoisotopic mass of 388.128571 Da .
Synthesis Analysis
Tetraphenylthiophene-based polyazomethines have been synthesized through a series of steps. The process involves the polycondensation of a new diamine, TPTPThDA, with proportionate aromatic dialdehydes . Another synthesis method involves a two-step thermal imidization derived from BATPDF with various commercially available dianhydrides .Molecular Structure Analysis
The molecular structure of Tetraphenylthiophene is based on a tetraphenylthiophene-core . It has been found that these molecules can form square, rectangular, or hexagonal columnar phases depending on the mesogenic core structures and the chain length of aliphatic tails .Chemical Reactions Analysis
Tetraphenylthiophene has been used in the synthesis of various polyazomethines . It has also been used in the creation of a covalent triazine-based framework .Physical And Chemical Properties Analysis
Tetraphenylthiophene has a density of 1.1±0.1 g/cm3, a boiling point of 402.2±14.0 °C at 760 mmHg, and a vapour pressure of 0.0±0.9 mmHg at 25°C . It also has an enthalpy of vaporization of 62.8±3.0 kJ/mol and a flash point of 147.8±6.3 °C .Applications De Recherche Scientifique
Luminescent Liquid Crystals
Tetraphenylthiophene has been used in the synthesis of luminescent liquid crystal (LLC) materials . These materials exhibit outstanding solid-state luminescence properties and have potential applications as semiconductor materials, liquid crystal displays, and organic light-emitting diodes . The fluorescence emission intensity values measured in the aggregated state were hundred times higher than those values measured in benign solvents .
Aggregation-Induced Emission
Tetraphenylthiophene exhibits aggregation-induced emission (AIE) characteristics . This property is significant because it opens up new possibilities for applications in modern technologies, such as photonics, optoelectronic devices, biological probes, and fluorescent sensors .
Organic Semiconductor Materials
The AIE-active tetraphenylthiophene core LLC materials showed narrow band gap energies . This property might make them suitable for use as organic semiconductor materials .
Sensors
The excellent gelation ability of these AIE-active tetraphenylthiophene core LLC materials in appropriate solvents suggests that they might have potential applications as sensors .
Optoelectronic Devices
The enhanced emission in the condensed phase (AIE) of tetraphenylthiophene and its potential use in optoelectronic devices have received much attention .
Biological Probes
The AIE property of tetraphenylthiophene can be utilized in the development of biological probes .
Safety and Hazards
Orientations Futures
Mécanisme D'action
Target of Action
Tetraphenylthiophene, also known as 2,3,4,5-Tetraphenylthiophene, is a propeller-shaped molecule that primarily targets the excited state mechanisms . It is a member of the family of molecules that exhibit Aggregation Induced Emission (AIE), although it features weaker AIE compared to other members .
Mode of Action
The interaction of Tetraphenylthiophene with its targets involves the excited state mechanisms and the role of triplet states . The nonradiative decay in Tetraphenylthiophene is driven by bond breaking, and due to the significant spin-orbit couplings along the reaction coordinate, intersystem crossing plays an important role in the mechanism .
Biochemical Pathways
The biochemical pathways affected by Tetraphenylthiophene involve the potential energy surfaces with a special focus on the role of triplet states . The aggregation in the solid state hampers the access to internal conversion pathways. Intersystem crossing is active in the crystal phase, which explains the weak aie of this molecule .
Pharmacokinetics
Its physical properties such as density (1142±006 g/cm3), melting point (184-185°C), boiling point (4022±140 °C), and vapor pressure (258E-06mmHg at 25°C) have been reported . These properties can influence its bioavailability and pharmacokinetics.
Result of Action
The molecular and cellular effects of Tetraphenylthiophene’s action are primarily related to its luminescent properties . It exhibits Aggregation Induced Emission (AIE) characteristics, and its fluorescence emission intensity values measured in the aggregated state were hundred times higher than those values measured in benign solvents .
Propriétés
IUPAC Name |
2,3,4,5-tetraphenylthiophene | |
---|---|---|
Source | PubChem | |
URL | https://pubchem.ncbi.nlm.nih.gov | |
Description | Data deposited in or computed by PubChem | |
InChI |
InChI=1S/C28H20S/c1-5-13-21(14-6-1)25-26(22-15-7-2-8-16-22)28(24-19-11-4-12-20-24)29-27(25)23-17-9-3-10-18-23/h1-20H | |
Source | PubChem | |
URL | https://pubchem.ncbi.nlm.nih.gov | |
Description | Data deposited in or computed by PubChem | |
InChI Key |
MQFBWJOMLIHUDY-UHFFFAOYSA-N | |
Source | PubChem | |
URL | https://pubchem.ncbi.nlm.nih.gov | |
Description | Data deposited in or computed by PubChem | |
Canonical SMILES |
C1=CC=C(C=C1)C2=C(SC(=C2C3=CC=CC=C3)C4=CC=CC=C4)C5=CC=CC=C5 | |
Source | PubChem | |
URL | https://pubchem.ncbi.nlm.nih.gov | |
Description | Data deposited in or computed by PubChem | |
Molecular Formula |
C28H20S | |
Source | PubChem | |
URL | https://pubchem.ncbi.nlm.nih.gov | |
Description | Data deposited in or computed by PubChem | |
DSSTOX Substance ID |
DTXSID90172189 | |
Record name | Tetraphenylthiophene | |
Source | EPA DSSTox | |
URL | https://comptox.epa.gov/dashboard/DTXSID90172189 | |
Description | DSSTox provides a high quality public chemistry resource for supporting improved predictive toxicology. | |
Molecular Weight |
388.5 g/mol | |
Source | PubChem | |
URL | https://pubchem.ncbi.nlm.nih.gov | |
Description | Data deposited in or computed by PubChem | |
Product Name |
Tetraphenylthiophene | |
CAS RN |
1884-68-0 | |
Record name | 2,3,4,5-Tetraphenylthiophene | |
Source | CAS Common Chemistry | |
URL | https://commonchemistry.cas.org/detail?cas_rn=1884-68-0 | |
Description | CAS Common Chemistry is an open community resource for accessing chemical information. Nearly 500,000 chemical substances from CAS REGISTRY cover areas of community interest, including common and frequently regulated chemicals, and those relevant to high school and undergraduate chemistry classes. This chemical information, curated by our expert scientists, is provided in alignment with our mission as a division of the American Chemical Society. | |
Explanation | The data from CAS Common Chemistry is provided under a CC-BY-NC 4.0 license, unless otherwise stated. | |
Record name | Tetraphenylthiophene | |
Source | ChemIDplus | |
URL | https://pubchem.ncbi.nlm.nih.gov/substance/?source=chemidplus&sourceid=0001884680 | |
Description | ChemIDplus is a free, web search system that provides access to the structure and nomenclature authority files used for the identification of chemical substances cited in National Library of Medicine (NLM) databases, including the TOXNET system. | |
Record name | Tetraphenylthiophene | |
Source | DTP/NCI | |
URL | https://dtp.cancer.gov/dtpstandard/servlet/dwindex?searchtype=NSC&outputformat=html&searchlist=56485 | |
Description | The NCI Development Therapeutics Program (DTP) provides services and resources to the academic and private-sector research communities worldwide to facilitate the discovery and development of new cancer therapeutic agents. | |
Explanation | Unless otherwise indicated, all text within NCI products is free of copyright and may be reused without our permission. Credit the National Cancer Institute as the source. | |
Record name | Tetraphenylthiophene | |
Source | EPA DSSTox | |
URL | https://comptox.epa.gov/dashboard/DTXSID90172189 | |
Description | DSSTox provides a high quality public chemistry resource for supporting improved predictive toxicology. | |
Record name | Tetraphenylthiophene | |
Source | European Chemicals Agency (ECHA) | |
URL | https://echa.europa.eu/substance-information/-/substanceinfo/100.015.951 | |
Description | The European Chemicals Agency (ECHA) is an agency of the European Union which is the driving force among regulatory authorities in implementing the EU's groundbreaking chemicals legislation for the benefit of human health and the environment as well as for innovation and competitiveness. | |
Explanation | Use of the information, documents and data from the ECHA website is subject to the terms and conditions of this Legal Notice, and subject to other binding limitations provided for under applicable law, the information, documents and data made available on the ECHA website may be reproduced, distributed and/or used, totally or in part, for non-commercial purposes provided that ECHA is acknowledged as the source: "Source: European Chemicals Agency, http://echa.europa.eu/". Such acknowledgement must be included in each copy of the material. ECHA permits and encourages organisations and individuals to create links to the ECHA website under the following cumulative conditions: Links can only be made to webpages that provide a link to the Legal Notice page. | |
Retrosynthesis Analysis
AI-Powered Synthesis Planning: Our tool employs the Template_relevance Pistachio, Template_relevance Bkms_metabolic, Template_relevance Pistachio_ringbreaker, Template_relevance Reaxys, Template_relevance Reaxys_biocatalysis model, leveraging a vast database of chemical reactions to predict feasible synthetic routes.
One-Step Synthesis Focus: Specifically designed for one-step synthesis, it provides concise and direct routes for your target compounds, streamlining the synthesis process.
Accurate Predictions: Utilizing the extensive PISTACHIO, BKMS_METABOLIC, PISTACHIO_RINGBREAKER, REAXYS, REAXYS_BIOCATALYSIS database, our tool offers high-accuracy predictions, reflecting the latest in chemical research and data.
Strategy Settings
Precursor scoring | Relevance Heuristic |
---|---|
Min. plausibility | 0.01 |
Model | Template_relevance |
Template Set | Pistachio/Bkms_metabolic/Pistachio_ringbreaker/Reaxys/Reaxys_biocatalysis |
Top-N result to add to graph | 6 |
Feasible Synthetic Routes
Q & A
Q1: What is the molecular formula and weight of Tetraphenylthiophene?
A1: Tetraphenylthiophene (TPT) has a molecular formula of C28H20S and a molecular weight of 388.53 g/mol.
Q2: What are the key spectroscopic characteristics of TPT?
A2: TPT can be characterized using various spectroscopic techniques, including:
Q3: Is TPT soluble in common organic solvents?
A3: TPT exhibits varying solubility depending on the specific solvent and the presence of substituents. While unsubstituted TPT has limited solubility, introducing flexible chains like nonanoyl groups or bulky substituents like 4-phenylquinoline can significantly improve its solubility in organic solvents such as tetrahydrofuran (THF), chloroform, and dichloromethane. [, , ]
Q4: How thermally stable is TPT?
A4: TPT generally demonstrates excellent thermal stability. Thermogravimetric analysis (TGA) indicates that polymers incorporating the TPT moiety often exhibit no weight loss below 400 °C, even in air. [, ] The specific thermal properties, such as glass transition temperature (Tg), can be influenced by the nature of the polymer backbone and the presence of other substituents. [, ]
Q5: Can TPT be incorporated into polymers, and how does this affect its properties?
A5: Yes, TPT has been successfully incorporated into various polymers, including polyimides, polyamides, and polyfluorenes. [, , , , , ] The inclusion of TPT often enhances the thermal stability and solubility of the resulting polymers. [, , ] Furthermore, the presence of the TPT moiety can influence the polymer's optical properties, potentially leading to applications in light-emitting diodes. [, , ]
Q6: What is aggregation-induced emission (AIE), and how does it relate to TPT?
A6: AIE is a phenomenon where certain molecules exhibit enhanced fluorescence emission upon aggregation. TPT and its derivatives have been extensively studied for their AIE properties. [, , , , , , , ] This behavior is often attributed to restricted intramolecular rotation (RIR) in the aggregated state, leading to a decrease in non-radiative decay pathways and an increase in fluorescence quantum yield. [, ]
Q7: How do substituents on TPT affect its AIE properties?
A7: The nature and position of substituents on the TPT core significantly impact its AIE behavior. Bulky substituents, like 4-phenylquinoline, can enhance RIR even in solution, leading to strong emission regardless of aggregation. [] Conversely, smaller substituents might require aggregation for pronounced AIE effects. [, ] The presence of hydrogen-bonding groups can further influence AIE by promoting intermolecular interactions and enhancing aggregation. []
Q8: Does TPT exhibit any catalytic activity?
A8: While TPT itself is not typically recognized as a catalyst, it plays a crucial role in understanding catalytic processes. For example, the hydrodesulfurization of sulfur-containing heterocycles, a significant reaction in petroleum refining, has been investigated using tetraphenylthiophene as a model compound. [] This research helps elucidate reaction mechanisms and the role of catalysts in removing sulfur from fossil fuels.
Q9: Have computational methods been used to study TPT?
A9: Yes, computational chemistry techniques, including Density Functional Theory (DFT) calculations, have been employed to investigate the electronic structure, optical properties, and piezo-responsive luminescence of TPT. [] These studies provide valuable insights into the relationship between molecular structure, packing arrangements, and the observed photophysical behavior of TPT.
Q10: How do structural modifications of TPT impact its properties?
A10: Modifying the TPT structure significantly affects its properties. Introducing electron-donating or electron-withdrawing groups can alter the electronic structure and influence its optical properties, such as absorption and emission wavelengths. [, ] Additionally, the size and flexibility of substituents play a crucial role in determining solubility, thermal stability, and aggregation behavior. [, , ]
Avertissement et informations sur les produits de recherche in vitro
Veuillez noter que tous les articles et informations sur les produits présentés sur BenchChem sont destinés uniquement à des fins informatives. Les produits disponibles à l'achat sur BenchChem sont spécifiquement conçus pour des études in vitro, qui sont réalisées en dehors des organismes vivants. Les études in vitro, dérivées du terme latin "in verre", impliquent des expériences réalisées dans des environnements de laboratoire contrôlés à l'aide de cellules ou de tissus. Il est important de noter que ces produits ne sont pas classés comme médicaments et n'ont pas reçu l'approbation de la FDA pour la prévention, le traitement ou la guérison de toute condition médicale, affection ou maladie. Nous devons souligner que toute forme d'introduction corporelle de ces produits chez les humains ou les animaux est strictement interdite par la loi. Il est essentiel de respecter ces directives pour assurer la conformité aux normes légales et éthiques en matière de recherche et d'expérimentation.