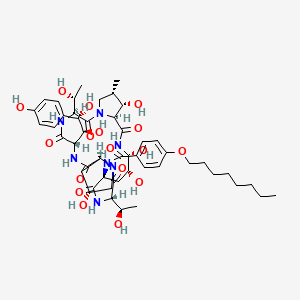
Cilofungine
Vue d'ensemble
Description
La cilofungine est le premier membre de la famille des antifongiques échinocandines à être appliqué en clinique. Elle a été dérivée d'un champignon du genre Aspergillus. La this compound interfère avec la capacité d'un champignon envahisseur à synthétiser la paroi cellulaire en inhibant la synthèse du (1→3)-β-D-glucane .
Applications De Recherche Scientifique
Introduction to Cilofungin
Cilofungin, a semisynthetic derivative of echinocandin B, was developed as an antifungal agent with the aim of treating invasive fungal infections. Despite its initial promise, clinical development faced significant challenges, leading to its withdrawal from Phase II trials due to nephrotoxicity and poor solubility. Nevertheless, cilofungin's mechanism of action and its inhibition of fungal cell wall synthesis make it a subject of continued interest in antifungal research.
Inhibition Studies
- In vitro Efficacy : Cilofungin has shown potent activity against various fungal pathogens, including Candida albicans and Aspergillus fumigatus. Studies indicate that cilofungin can inhibit (1,3)-beta-D-glucan synthase at concentrations as low as 0.19 micrograms/milliliter, demonstrating its potential effectiveness in clinical settings .
- Dose-Response Relationships : Research has established that cilofungin's inhibitory effects are dose-dependent, with maximal inhibition observed at approximately 1.25 micrograms/milliliter .
Clinical Applications
Although cilofungin itself was not successfully developed for clinical use, its study has contributed to the understanding and development of other echinocandins currently used in clinical practice.
Comparative Analysis with Other Echinocandins
Echinocandin | Mechanism | Clinical Use | Withdrawn Status |
---|---|---|---|
Cilofungin | Inhibits (1,3)-beta-D-glucan synthase | None (development halted) | Yes |
Anidulafungin | Inhibits (1,3)-beta-D-glucan synthase | Candidemia, invasive candidiasis | No |
Caspofungin | Inhibits (1,3)-beta-D-glucan synthase | Aspergillosis, candidemia | No |
This table illustrates the position of cilofungin within the broader context of echinocandin drugs.
Case Study Insights
- In Vitro Activity Against Clinical Isolates : Cilofungin has been tested against 256 clinical isolates of yeasts, showing significant antifungal activity. The minimum inhibitory concentration (MIC) for 90% of isolates was notably low for Candida albicans, indicating its potential effectiveness against common fungal infections .
- Influence of pH on Activity : Studies have shown that pH significantly affects cilofungin's antifungal activity. Optimal pH levels enhance its efficacy against pathogenic yeasts .
- Pharmacokinetics and Toxicity : Investigations into cilofungin’s pharmacokinetics revealed challenges related to nephrotoxicity and solubility in clinical formulations. These factors contributed to its discontinuation in clinical trials despite promising initial results .
Mécanisme D'action
Cilofungin, also known as Cilofungina, is a member of the echinocandin family of antifungal drugs . This article will cover the mechanism of action of Cilofungin, including its primary targets, mode of action, biochemical pathways, pharmacokinetics, the results of its action, and the influence of environmental factors on its action.
Target of Action
Cilofungin primarily targets the 1,3-beta-glucan synthase , an enzyme crucial for the synthesis of the fungal cell wall . This enzyme is responsible for the production of (1→3)-β-D-glucan, a major component of the fungal cell wall .
Mode of Action
Cilofungin interacts with its target, the 1,3-beta-glucan synthase, by inhibiting its activity . This inhibition interferes with the fungus’s ability to synthesize its cell wall, specifically disrupting the production of (1→3)-β-D-glucan .
Biochemical Pathways
The primary biochemical pathway affected by Cilofungin is the synthesis of the fungal cell wall . By inhibiting 1,3-beta-glucan synthase, Cilofungin disrupts the production of (1→3)-β-D-glucan, a key component of the fungal cell wall . This disruption can lead to severe modifications of the cell wall and cytoplasmic membrane of sensitive organisms .
Pharmacokinetics
The pharmacokinetics of Cilofungin are characterized by a short plasma half-life and saturable nonlinear plasma pharmacokinetics . At lower dosage regimens, Cilofungin follows linear plasma pharmacokinetics, whereas at higher doses, nonlinear kinetics consistent with a saturated elimination pathway(s) are observed .
Result of Action
The inhibition of 1,3-beta-glucan synthase by Cilofungin leads to a disruption in the synthesis of the fungal cell wall . This disruption can result in severe modifications of the cell wall and cytoplasmic membrane of sensitive organisms , leading to the death of the fungus.
Action Environment
The efficacy of Cilofungin can be influenced by environmental factors such as pH . As the pH decreases from 7.4 to 3.0, the inhibitory concentrations of Cilofungin can increase up to 64-fold . This suggests that the action, efficacy, and stability of Cilofungin can be significantly affected by the pH of the environment .
Analyse Biochimique
Biochemical Properties
Cilofungin plays a crucial role in biochemical reactions by targeting and inhibiting the enzyme (1,3)-β-D-glucan synthase. This enzyme is responsible for the synthesis of (1→3)-β-D-glucan, a key structural component of the fungal cell wall. By inhibiting this enzyme, Cilofungin disrupts the formation of the cell wall, leading to cell lysis and death. The compound interacts specifically with the glucan synthase complex, binding to the enzyme and preventing the polymerization of glucose monomers into the glucan polymer .
Cellular Effects
Cilofungin exerts significant effects on various types of cells, particularly fungal cells. It disrupts cell wall synthesis, leading to structural modifications in the cell wall and cytoplasmic membrane. In Candida albicans, exposure to Cilofungin results in cracks and discontinuities in the cell wall, undulation and fracturing of the cell membrane, and the release of cellular components. These changes inhibit cell division and germ tube formation, ultimately leading to cell death . Additionally, Cilofungin’s inhibition of (1,3)-β-D-glucan synthase affects cell signaling pathways and gene expression related to cell wall synthesis and maintenance .
Molecular Mechanism
The molecular mechanism of Cilofungin involves the inhibition of (1,3)-β-D-glucan synthase, an enzyme critical for fungal cell wall synthesis. Cilofungin binds to the enzyme, preventing the polymerization of glucose monomers into (1→3)-β-D-glucan. This inhibition disrupts the structural integrity of the fungal cell wall, leading to cell lysis and death. The binding interaction between Cilofungin and the glucan synthase complex is highly specific, ensuring targeted action against fungal cells while minimizing effects on mammalian cells .
Temporal Effects in Laboratory Settings
In laboratory settings, the effects of Cilofungin have been observed to change over time. The compound exhibits stability in vitro, maintaining its antifungal activity over extended periods. Its efficacy can be influenced by factors such as concentration and exposure duration. Long-term exposure to Cilofungin in in vitro studies has shown sustained inhibition of fungal growth and cell wall synthesis . In vivo studies have demonstrated that Cilofungin’s antifungal activity correlates with its inhibitory effects on glucan synthase, with effective doses leading to significant reductions in fungal burden .
Dosage Effects in Animal Models
The effects of Cilofungin vary with different dosages in animal models. Studies have shown that higher doses of Cilofungin result in greater inhibition of fungal growth and more pronounced disruption of cell wall integrity. At high doses, Cilofungin can exhibit toxic effects, including nephrotoxicity, which led to the discontinuation of its clinical trials . Threshold effects have been observed, with specific dosages required to achieve effective antifungal activity without causing adverse effects .
Metabolic Pathways
Cilofungin is involved in metabolic pathways related to its antifungal activity. The compound interacts with enzymes and cofactors involved in the synthesis of (1→3)-β-D-glucan, disrupting the normal metabolic flux and leading to the accumulation of intermediate metabolites. This disruption affects the overall metabolic balance within fungal cells, contributing to their death . The metabolic pathways influenced by Cilofungin are primarily related to cell wall synthesis and maintenance .
Transport and Distribution
Within cells and tissues, Cilofungin is transported and distributed through interactions with specific transporters and binding proteins. The compound’s lipophilic nature facilitates its uptake and distribution within fungal cells, allowing it to reach its target site, the glucan synthase complex . Cilofungin’s distribution is influenced by factors such as concentration gradients and cellular localization, ensuring effective inhibition of cell wall synthesis .
Subcellular Localization
Cilofungin’s subcellular localization is primarily within the fungal cell wall and cytoplasmic membrane. The compound targets the glucan synthase complex, which is localized in the cell membrane, and inhibits its activity, leading to structural modifications in the cell wall . The specific targeting of Cilofungin to the cell wall and membrane ensures its effectiveness in disrupting fungal cell wall synthesis while minimizing effects on other cellular compartments .
Méthodes De Préparation
La cilofungine est un analogue semi-synthétique de l'échinocandine B. La préparation implique la fermentation d'espèces d'Aspergillus pour produire de l'échinocandine B, suivie de modifications chimiques pour introduire une chaîne latérale 4-octyloxybenzoate . Les méthodes de production industrielle comprennent la fermentation, la mutagenèse classique, le marquage isotopique et la synthèse chimique .
Analyse Des Réactions Chimiques
La cilofungine subit diverses réactions chimiques, notamment :
Oxydation : La this compound peut être oxydée dans des conditions spécifiques, conduisant à la formation de différents produits oxydés.
Réduction : Les réactions de réduction peuvent modifier les groupes fonctionnels présents dans la this compound.
Substitution : Des réactions de substitution peuvent se produire à diverses positions sur la molécule, conduisant à la formation de dérivés ayant des propriétés différentes.
Les réactifs et les conditions courants utilisés dans ces réactions comprennent des agents oxydants comme le peroxyde d'hydrogène, des agents réducteurs comme le borohydrure de sodium et divers catalyseurs pour les réactions de substitution. Les principaux produits formés à partir de ces réactions dépendent des conditions et des réactifs spécifiques utilisés .
Comparaison Avec Des Composés Similaires
La cilofungine est comparée à d'autres échinocandines telles que la caspofungine, la micafungine et l'anidulafungine. Ces composés partagent un mécanisme d'action similaire, mais diffèrent par leur structure chimique et leurs propriétés pharmacologiques. La this compound est unique en raison de ses modifications spécifiques de la chaîne latérale, qui réduisent son activité hémolytique tout en conservant ses propriétés antifongiques .
Des composés similaires comprennent :
- Caspofungine
- Micafungine
- Anidulafungine
- Amphotéricine B
- Kéconazole
Ces composés sont utilisés dans le traitement des infections fongiques et ont des degrés d'efficacité et de toxicité variables .
Propriétés
Numéro CAS |
79404-91-4 |
---|---|
Formule moléculaire |
C49H71N7O17 |
Poids moléculaire |
1030.1 g/mol |
Nom IUPAC |
N-[(3S,6S,9S,11R,15S,20R,21R,24S,25S,26S)-6-[(1S,2S)-1,2-dihydroxy-2-(4-hydroxyphenyl)ethyl]-11,20,21,25-tetrahydroxy-3,15-bis[(1R)-1-hydroxyethyl]-26-methyl-2,5,8,14,17,23-hexaoxo-1,4,7,13,16,22-hexazatricyclo[22.3.0.09,13]heptacosan-18-yl]-4-octoxybenzamide |
InChI |
InChI=1S/C49H71N7O17/c1-5-6-7-8-9-10-19-73-31-17-13-28(14-18-31)42(65)50-32-21-34(61)45(68)54-47(70)38-39(62)24(2)22-56(38)49(72)36(26(4)58)52-46(69)37(41(64)40(63)27-11-15-29(59)16-12-27)53-44(67)33-20-30(60)23-55(33)48(71)35(25(3)57)51-43(32)66/h11-18,24-26,30,32-41,45,57-64,68H,5-10,19-23H2,1-4H3,(H,50,65)(H,51,66)(H,52,69)(H,53,67)(H,54,70)/t24-,25+,26+,30+,32?,33-,34+,35-,36-,37-,38-,39-,40-,41-,45+/m0/s1 |
Clé InChI |
ZKZKCEAHVFVZDJ-HFNPJXHZSA-N |
SMILES |
CCCCCCCCOC1=CC=C(C=C1)C(=O)NC2CC(C(NC(=O)C3C(C(CN3C(=O)C(NC(=O)C(NC(=O)C4CC(CN4C(=O)C(NC2=O)C(C)O)O)C(C(C5=CC=C(C=C5)O)O)O)C(C)O)C)O)O)O |
SMILES isomérique |
CCCCCCCCOC1=CC=C(C=C1)C(=O)NC2C[C@H]([C@H](NC(=O)[C@@H]3[C@H]([C@H](CN3C(=O)[C@@H](NC(=O)[C@@H](NC(=O)[C@@H]4C[C@H](CN4C(=O)[C@@H](NC2=O)[C@@H](C)O)O)[C@@H]([C@H](C5=CC=C(C=C5)O)O)O)[C@@H](C)O)C)O)O)O |
SMILES canonique |
CCCCCCCCOC1=CC=C(C=C1)C(=O)NC2CC(C(NC(=O)C3C(C(CN3C(=O)C(NC(=O)C(NC(=O)C4CC(CN4C(=O)C(NC2=O)C(C)O)O)C(C(C5=CC=C(C=C5)O)O)O)C(C)O)C)O)O)O |
Apparence |
Solid powder |
Pureté |
>98% (or refer to the Certificate of Analysis) |
Durée de conservation |
>3 years if stored properly |
Solubilité |
Soluble in DMSO |
Stockage |
Dry, dark and at 0 - 4 C for short term (days to weeks) or -20 C for long term (months to years). |
Synonymes |
cilofungin L 646991 L-646,991 LY 121019 LY-121019 |
Origine du produit |
United States |
Retrosynthesis Analysis
AI-Powered Synthesis Planning: Our tool employs the Template_relevance Pistachio, Template_relevance Bkms_metabolic, Template_relevance Pistachio_ringbreaker, Template_relevance Reaxys, Template_relevance Reaxys_biocatalysis model, leveraging a vast database of chemical reactions to predict feasible synthetic routes.
One-Step Synthesis Focus: Specifically designed for one-step synthesis, it provides concise and direct routes for your target compounds, streamlining the synthesis process.
Accurate Predictions: Utilizing the extensive PISTACHIO, BKMS_METABOLIC, PISTACHIO_RINGBREAKER, REAXYS, REAXYS_BIOCATALYSIS database, our tool offers high-accuracy predictions, reflecting the latest in chemical research and data.
Strategy Settings
Precursor scoring | Relevance Heuristic |
---|---|
Min. plausibility | 0.01 |
Model | Template_relevance |
Template Set | Pistachio/Bkms_metabolic/Pistachio_ringbreaker/Reaxys/Reaxys_biocatalysis |
Top-N result to add to graph | 6 |
Feasible Synthetic Routes
Q1: What is the primary target of cilofungin in fungal cells?
A1: Cilofungin targets (1,3)-β-D-glucan synthase, a key enzyme responsible for the synthesis of (1,3)-β-D-glucan in the fungal cell wall. [, , ]
Q2: How does cilofungin inhibit (1,3)-β-D-glucan synthase?
A2: Cilofungin acts as a noncompetitive inhibitor of (1,3)-β-D-glucan synthase, binding to the enzyme at a site distinct from the substrate binding site. [, ]
Q3: What are the downstream effects of cilofungin's inhibition of (1,3)-β-D-glucan synthase?
A3: Inhibition of (1,3)-β-D-glucan synthase disrupts the synthesis of (1,3)-β-D-glucan, a critical structural component of the fungal cell wall. This disruption weakens the cell wall, ultimately leading to fungal cell death. [, , ]
Q4: What is the molecular formula and weight of cilofungin?
A4: Due to the proprietary nature of cilofungin's development, the exact molecular formula and weight are not readily available in the provided research papers.
Q5: Is there spectroscopic data available for cilofungin?
A6: While the provided research papers do not delve into detailed spectroscopic analysis, one study utilized cilofungin's inherent fluorescence properties to investigate its interaction with fungal membranes. [] This suggests that fluorescence spectroscopy might be a viable technique for studying cilofungin.
Q6: What is the role of the lipophilic side chain in cilofungin's activity?
A7: The lipophilic side chain of cilofungin is essential for its antifungal activity and its ability to inhibit (1,3)-β-D-glucan synthase. Studies have shown that removing or modifying this side chain significantly reduces or abolishes its activity. [, ]
Q7: How does modifying the cyclic peptide core of cilofungin affect its activity?
A8: Research utilizing simplified cilofungin analogs, synthesized through solid-phase methods, revealed that structural complexity in the cyclic peptide core is crucial for antifungal activity. Notably, the 3-hydroxy-4-methylproline residue appears to enhance activity, while the L-homotyrosine residue is essential for both antifungal activity and (1,3)-β-D-glucan synthase inhibition. []
Q8: How is cilofungin administered, and what is its pharmacokinetic profile?
A9: Cilofungin has been investigated for intravenous administration in animal models. Studies in rabbits revealed that its pharmacokinetics are nonlinear and saturable, meaning its clearance decreases as the dose increases. [] This leads to higher than expected plasma concentrations with continuous infusion compared to intermittent dosing.
Q9: What is the relationship between cilofungin's pharmacokinetics and its in vivo antifungal activity?
A11: Studies in rabbits with disseminated candidiasis found a direct correlation between tissue concentrations of cilofungin and antifungal activity. Dosage regimens that achieved higher tissue concentrations, particularly those utilizing continuous infusion, exhibited greater efficacy in reducing fungal burden in various organs. []
Q10: Which Candida species are most susceptible to cilofungin in vitro?
A12: Cilofungin exhibits potent in vitro activity against Candida albicans and Candida tropicalis. [, , , , , , , , ]
Q11: What is cilofungin's in vitro activity against other Candida species and non-Candida fungi?
A13: Cilofungin shows less potent or limited in vitro activity against other Candida species such as Candida glabrata, Candida krusei, Candida parapsilosis, and Candida guilliermondii. [, , , , , , ] It also demonstrates limited activity against Aspergillus fumigatus, Cryptococcus neoformans, Blastomyces dermatitidis, and other non-Candida fungi. [, , , , ]
Q12: What about cilofungin's efficacy in other animal models of fungal infections?
A15: While cilofungin shows promise against Candida infections, it has been less successful in animal models of other fungal diseases. For instance, it failed to demonstrate efficacy in a rabbit model of Candida albicans endocarditis [] and showed antagonistic effects when combined with amphotericin B in a murine model of disseminated aspergillosis. []
Q13: Are there known mechanisms of resistance to cilofungin?
A16: Research suggests that cilofungin resistance can arise through alterations in the target enzyme (1,3)-β-D-glucan synthase. One study utilizing a cilofungin-resistant Candida albicans strain found that while the drug initially inhibited glucan synthesis, the resistant strain could eventually overcome this inhibition and resume normal growth. [] This suggests the existence of adaptive mechanisms that allow some Candida species to bypass cilofungin's inhibitory effects.
Avertissement et informations sur les produits de recherche in vitro
Veuillez noter que tous les articles et informations sur les produits présentés sur BenchChem sont destinés uniquement à des fins informatives. Les produits disponibles à l'achat sur BenchChem sont spécifiquement conçus pour des études in vitro, qui sont réalisées en dehors des organismes vivants. Les études in vitro, dérivées du terme latin "in verre", impliquent des expériences réalisées dans des environnements de laboratoire contrôlés à l'aide de cellules ou de tissus. Il est important de noter que ces produits ne sont pas classés comme médicaments et n'ont pas reçu l'approbation de la FDA pour la prévention, le traitement ou la guérison de toute condition médicale, affection ou maladie. Nous devons souligner que toute forme d'introduction corporelle de ces produits chez les humains ou les animaux est strictement interdite par la loi. Il est essentiel de respecter ces directives pour assurer la conformité aux normes légales et éthiques en matière de recherche et d'expérimentation.