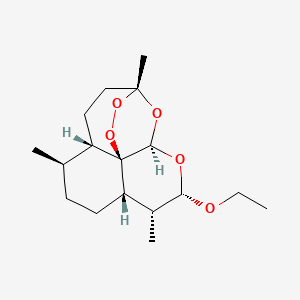
Artéméther
Vue d'ensemble
Description
Applications De Recherche Scientifique
Artemotil has several scientific research applications, including:
Anti-malarial: It is primarily used for the treatment of chloroquine-resistant Plasmodium falciparum malaria and cerebral malaria.
Anti-inflammatory: Artemotil has been found to alleviate signs of colitis by increasing the expansion of Type 1 regulatory T cells and inhibiting the generation of Th1 and Th17 cells.
Mécanisme D'action
Target of Action
Arteether, like other artemisinin derivatives, primarily targets the erythrocytic stages of Plasmodium species, including Plasmodium falciparum . The precise primary target of artemisinin and its derivatives, including arteether, remains unclear .
Mode of Action
Arteether is a schizonticidal drug. It contains endoperoxide bridges that are cleaved upon binding with haeme, a byproduct of hemoglobin degradation . This cleavage liberates highly active free radicals that bind to membrane proteins, causing lipid peroxidation and damaging the endoplasmic reticulum . This process inhibits protein synthesis, leading to the lysis of the parasite .
Biochemical Pathways
Arteether, like other artemisinin derivatives, generates reactive oxygen species (ROS) and free radicals within the malaria parasite . These radicals may target essential parasite macromolecules, causing the parasite’s death . The endoperoxide bond in arteether could be activated by reduced heme or ferrous iron, leading to the production of cytotoxic carbon-centered radicals .
Pharmacokinetics
Arteether is metabolized into the active metabolite dihydroartemisinin (DHA) in the body . The pharmacokinetics and bioavailability of arteether have been investigated in rats after single intravenous, intramuscular, and intragastric doses . After oral administration, low bioavailability (19–35%) was observed for all five drugs .
Result of Action
The action of arteether results in the reduction of the number of malarial parasites, providing rapid symptomatic relief . Its anti-proliferation effects have been observed mostly in Hepatocellular Carcinoma (HCC) cell lines . DHA, the active metabolite of arteether, inhibited the proliferation of human HCC cells in a dose- and time-dependent manner .
Action Environment
The efficacy of arteether, like other artemisinin derivatives, can be influenced by various environmental factors. For instance, the rate and extent of development of resistance to artemisinin-based drugs will determine their future use . Better understanding of mechanisms of action and resistance and policy initiatives to prevent or delay resistance will be crucial .
Analyse Biochimique
Biochemical Properties
Arteether plays a crucial role in biochemical reactions by interacting with various biomolecules within the malaria parasite. The compound is known to interact with heme, a component of hemoglobin, within the parasite’s digestive vacuole. The interaction between arteether and heme leads to the generation of reactive oxygen species, which cause oxidative damage to the parasite’s cellular components . Additionally, arteether has been shown to inhibit the activity of several enzymes involved in the parasite’s metabolic pathways, further contributing to its antimalarial effects .
Cellular Effects
Arteether exerts significant effects on various types of cells, particularly those of the malaria parasite. It disrupts the parasite’s cellular processes by inducing oxidative stress, leading to the accumulation of reactive oxygen species. This oxidative stress damages the parasite’s cellular membranes, proteins, and DNA, ultimately resulting in cell death . Arteether also affects the host’s immune cells by modulating the production of cytokines, which are signaling molecules that regulate immune responses .
Molecular Mechanism
The molecular mechanism of arteether involves several key interactions at the molecular level. Arteether binds to heme within the parasite’s digestive vacuole, leading to the cleavage of its peroxide bond and the generation of reactive oxygen species . These reactive oxygen species cause oxidative damage to the parasite’s cellular components, including lipids, proteins, and nucleic acids. Additionally, arteether inhibits the activity of enzymes involved in the parasite’s metabolic pathways, such as the electron transport chain and glycolysis, further contributing to its antimalarial effects .
Temporal Effects in Laboratory Settings
In laboratory settings, the effects of arteether have been observed to change over time. Arteether is known for its stability, with a relatively long shelf life under proper storage conditions . Its efficacy can decrease over time due to degradation, particularly when exposed to light and heat. Long-term studies have shown that arteether can have sustained antimalarial effects, but its potency may diminish with prolonged exposure to adverse conditions .
Dosage Effects in Animal Models
The effects of arteether vary with different dosages in animal models. At therapeutic doses, arteether effectively clears malaria parasites from the bloodstream without causing significant adverse effects . At higher doses, arteether can induce toxicity, leading to adverse effects such as neurotoxicity and hepatotoxicity . Threshold effects have been observed, where increasing the dosage beyond a certain point does not significantly enhance its antimalarial efficacy but increases the risk of toxicity .
Metabolic Pathways
Arteether is involved in several metabolic pathways within the malaria parasite. It interacts with enzymes such as cytochrome P450, which is involved in the metabolism of various endogenous and exogenous compounds . Arteether’s interaction with these enzymes can affect metabolic flux and alter the levels of metabolites within the parasite. Additionally, arteether’s metabolism in the host involves its conversion to dihydroartemisinin, an active metabolite that also exhibits antimalarial activity .
Transport and Distribution
Arteether is transported and distributed within cells and tissues through various mechanisms. It is highly lipophilic, allowing it to easily cross cellular membranes and accumulate within the parasite’s digestive vacuole . Arteether interacts with transporters and binding proteins that facilitate its uptake and distribution within the parasite and host cells. Its lipophilicity also contributes to its accumulation in fatty tissues, which can affect its overall distribution and efficacy .
Subcellular Localization
The subcellular localization of arteether is primarily within the digestive vacuole of the malaria parasite. This localization is crucial for its antimalarial activity, as it allows arteether to interact with heme and generate reactive oxygen species . Arteether’s activity and function are influenced by its localization, with targeting signals and post-translational modifications directing it to specific compartments within the parasite. These modifications ensure that arteether exerts its effects precisely where they are needed to combat the malaria parasite .
Méthodes De Préparation
L'artemotil est synthétisé à partir de l'artémisinine par une série de réactions chimiques. Le procédé implique la réduction de l'artémisinine en dihydroartémisinine, suivie d'une éthérification pour produire l'artemotil . La production industrielle de l'artemotil implique généralement les étapes suivantes :
Réduction de l'artémisinine : L'artémisinine est réduite en dihydroartémisinine à l'aide d'un agent réducteur tel que le borohydrure de sodium.
Éthérification : La dihydroartémisinine est ensuite mise à réagir avec de l'éthanol en présence d'un catalyseur acide pour former de l'artemotil.
Analyse Des Réactions Chimiques
L'artemotil subit diverses réactions chimiques, notamment :
Oxydation : L'artemotil peut être oxydé pour former des dérivés de l'artémisinine.
Réduction : Il peut être réduit en dihydroartémisinine.
Substitution : L'artemotil peut subir des réactions de substitution où le groupe éthoxy est remplacé par d'autres groupes fonctionnels.
Les réactifs et les conditions courantes utilisés dans ces réactions comprennent :
Agents oxydants : tels que le peroxyde d'hydrogène ou le permanganate de potassium.
Agents réducteurs : tels que le borohydrure de sodium.
Catalyseurs acides : tels que l'acide sulfurique ou l'acide chlorhydrique.
Les principaux produits formés à partir de ces réactions comprennent divers dérivés de l'artémisinine et la dihydroartémisinine .
4. Applications de la recherche scientifique
L'artemotil a plusieurs applications de recherche scientifique, notamment :
Antipaludique : Il est principalement utilisé pour le traitement du paludisme à Plasmodium falciparum résistant à la chloroquine et du paludisme cérébral.
Anti-inflammatoire : L'artemotil a été trouvé pour atténuer les signes de colite en augmentant l'expansion des cellules T régulatrices de type 1 et en inhibant la génération des cellules Th1 et Th17.
5. Mécanisme d'action
L'artemotil exerce ses effets en générant des espèces réactives de l'oxygène qui endommagent les composants cellulaires du parasite. Il cible spécifiquement les stades érythrocytaires de Plasmodium falciparum en inhibant la synthèse des acides nucléiques et des protéines . Les cibles moléculaires et les voies impliquées comprennent l'inhibition de la détoxification de l'hème et la perturbation de la fonction mitochondriale dans le parasite .
Comparaison Avec Des Composés Similaires
L'artemotil fait partie de la famille des composés de l'artémisinine, qui comprend l'artémisinine, la dihydroartémisinine, l'artéméther et l'artésunate . Comparé à ces composés, l'artemotil a une demi-vie plus longue et est plus liposoluble, ce qui permet une libération plus lente lorsqu'il est administré par voie intramusculaire . Cela le rend particulièrement utile dans les cas de paludisme grave où une action prolongée est bénéfique.
Composés similaires
Artésinine : Le composé parent, dérivé d'Artemisia annua.
Dihydroartémisinine : Une forme réduite de l'artémisinine.
This compound : Un dérivé méthyléther de la dihydroartémisinine.
Artésunate : Un dérivé hydrosoluble de la dihydroartémisinine.
L'unicité de l'artemotil réside dans sa liposolubilité et son action prolongée, ce qui en fait une option précieuse dans le traitement du paludisme grave .
Propriétés
IUPAC Name |
(1R,4S,5R,8S,9R,10S,12R,13R)-10-ethoxy-1,5,9-trimethyl-11,14,15,16-tetraoxatetracyclo[10.3.1.04,13.08,13]hexadecane | |
---|---|---|
Source | PubChem | |
URL | https://pubchem.ncbi.nlm.nih.gov | |
Description | Data deposited in or computed by PubChem | |
InChI |
InChI=1S/C17H28O5/c1-5-18-14-11(3)13-7-6-10(2)12-8-9-16(4)20-15(19-14)17(12,13)22-21-16/h10-15H,5-9H2,1-4H3/t10-,11-,12+,13+,14+,15-,16-,17-/m1/s1 | |
Source | PubChem | |
URL | https://pubchem.ncbi.nlm.nih.gov | |
Description | Data deposited in or computed by PubChem | |
InChI Key |
NLYNIRQVMRLPIQ-XQLAAWPRSA-N | |
Source | PubChem | |
URL | https://pubchem.ncbi.nlm.nih.gov | |
Description | Data deposited in or computed by PubChem | |
Canonical SMILES |
CCOC1C(C2CCC(C3C24C(O1)OC(CC3)(OO4)C)C)C | |
Source | PubChem | |
URL | https://pubchem.ncbi.nlm.nih.gov | |
Description | Data deposited in or computed by PubChem | |
Isomeric SMILES |
CCO[C@@H]1[C@@H]([C@@H]2CC[C@H]([C@H]3[C@]24[C@H](O1)O[C@@](CC3)(OO4)C)C)C | |
Source | PubChem | |
URL | https://pubchem.ncbi.nlm.nih.gov | |
Description | Data deposited in or computed by PubChem | |
Molecular Formula |
C17H28O5 | |
Source | PubChem | |
URL | https://pubchem.ncbi.nlm.nih.gov | |
Description | Data deposited in or computed by PubChem | |
Molecular Weight |
312.4 g/mol | |
Source | PubChem | |
URL | https://pubchem.ncbi.nlm.nih.gov | |
Description | Data deposited in or computed by PubChem | |
CAS No. |
75887-54-6 | |
Record name | Arteether | |
Source | CAS Common Chemistry | |
URL | https://commonchemistry.cas.org/detail?cas_rn=75887-54-6 | |
Description | CAS Common Chemistry is an open community resource for accessing chemical information. Nearly 500,000 chemical substances from CAS REGISTRY cover areas of community interest, including common and frequently regulated chemicals, and those relevant to high school and undergraduate chemistry classes. This chemical information, curated by our expert scientists, is provided in alignment with our mission as a division of the American Chemical Society. | |
Explanation | The data from CAS Common Chemistry is provided under a CC-BY-NC 4.0 license, unless otherwise stated. | |
Record name | Artemotil | |
Source | DrugBank | |
URL | https://www.drugbank.ca/drugs/DB13851 | |
Description | The DrugBank database is a unique bioinformatics and cheminformatics resource that combines detailed drug (i.e. chemical, pharmacological and pharmaceutical) data with comprehensive drug target (i.e. sequence, structure, and pathway) information. | |
Explanation | Creative Common's Attribution-NonCommercial 4.0 International License (http://creativecommons.org/licenses/by-nc/4.0/legalcode) | |
Record name | ARTEMOTIL | |
Source | FDA Global Substance Registration System (GSRS) | |
URL | https://gsrs.ncats.nih.gov/ginas/app/beta/substances/XGL7GFB9YI | |
Description | The FDA Global Substance Registration System (GSRS) enables the efficient and accurate exchange of information on what substances are in regulated products. Instead of relying on names, which vary across regulatory domains, countries, and regions, the GSRS knowledge base makes it possible for substances to be defined by standardized, scientific descriptions. | |
Explanation | Unless otherwise noted, the contents of the FDA website (www.fda.gov), both text and graphics, are not copyrighted. They are in the public domain and may be republished, reprinted and otherwise used freely by anyone without the need to obtain permission from FDA. Credit to the U.S. Food and Drug Administration as the source is appreciated but not required. | |
Retrosynthesis Analysis
AI-Powered Synthesis Planning: Our tool employs the Template_relevance Pistachio, Template_relevance Bkms_metabolic, Template_relevance Pistachio_ringbreaker, Template_relevance Reaxys, Template_relevance Reaxys_biocatalysis model, leveraging a vast database of chemical reactions to predict feasible synthetic routes.
One-Step Synthesis Focus: Specifically designed for one-step synthesis, it provides concise and direct routes for your target compounds, streamlining the synthesis process.
Accurate Predictions: Utilizing the extensive PISTACHIO, BKMS_METABOLIC, PISTACHIO_RINGBREAKER, REAXYS, REAXYS_BIOCATALYSIS database, our tool offers high-accuracy predictions, reflecting the latest in chemical research and data.
Strategy Settings
Precursor scoring | Relevance Heuristic |
---|---|
Min. plausibility | 0.01 |
Model | Template_relevance |
Template Set | Pistachio/Bkms_metabolic/Pistachio_ringbreaker/Reaxys/Reaxys_biocatalysis |
Top-N result to add to graph | 6 |
Feasible Synthetic Routes
Avertissement et informations sur les produits de recherche in vitro
Veuillez noter que tous les articles et informations sur les produits présentés sur BenchChem sont destinés uniquement à des fins informatives. Les produits disponibles à l'achat sur BenchChem sont spécifiquement conçus pour des études in vitro, qui sont réalisées en dehors des organismes vivants. Les études in vitro, dérivées du terme latin "in verre", impliquent des expériences réalisées dans des environnements de laboratoire contrôlés à l'aide de cellules ou de tissus. Il est important de noter que ces produits ne sont pas classés comme médicaments et n'ont pas reçu l'approbation de la FDA pour la prévention, le traitement ou la guérison de toute condition médicale, affection ou maladie. Nous devons souligner que toute forme d'introduction corporelle de ces produits chez les humains ou les animaux est strictement interdite par la loi. Il est essentiel de respecter ces directives pour assurer la conformité aux normes légales et éthiques en matière de recherche et d'expérimentation.