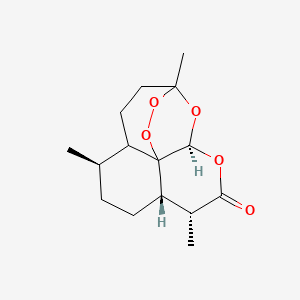
Artéméther
Vue d'ensemble
Description
Elle a été découverte en 1972 par Tu Youyou, qui a reçu le prix Nobel de physiologie ou médecine en 2015 pour cette découverte . L'artémisinine et ses dérivés sont réputés pour leurs puissantes propriétés antipaludiques, en particulier contre Plasmodium falciparum, le parasite responsable de la forme la plus grave du paludisme .
Applications De Recherche Scientifique
Artemisinin and its derivatives have a wide range of scientific research applications:
Mécanisme D'action
The mechanism of action of artemisinin involves the cleavage of its endoperoxide bridge by iron ions within the parasite’s cells. This reaction generates reactive oxygen species that damage vital proteins and membranes, leading to the death of the parasite . Artemisinin targets the schizont stage of the parasite’s life cycle, which is crucial for its rapid antimalarial effects .
Orientations Futures
Analyse Biochimique
Biochemical Properties
Artemisinin plays a crucial role in biochemical reactions, primarily through its interaction with heme and iron. The endoperoxide bridge in artemisinin is activated by reduced heme or ferrous iron, leading to the production of cytotoxic carbon-centered radicals . These radicals are highly potent alkylating agents that target essential macromolecules within parasites, leading to their death . Artemisinin interacts with several enzymes and proteins, including apoptosis-related proteins such as BAX, FASL, and caspase-3, as well as multi-drug resistance genes and cytokines like CD4+ and CD8+ . Additionally, artemisinin affects inflammation-related molecules such as NF-κB and COX2, telomerase, and oxidative stress molecules .
Cellular Effects
Artemisinin exerts significant effects on various types of cells and cellular processes. It has been shown to regulate the expression of proinflammatory and anti-inflammatory cytokines, influencing the activation and frequency of T helper and B cells, macrophages, dendritic cells, neutrophils, mast cells, and myeloid-derived suppressor cells . Artemisinin also affects cell signaling pathways, gene expression, and cellular metabolism. For instance, it can induce apoptosis, cause cell cycle arrest, and disrupt cancer invasion and metastasis . Furthermore, artemisinin influences the tumor microenvironment and mediates tumor-related signaling pathways .
Molecular Mechanism
The molecular mechanism of artemisinin involves the activation of its endoperoxide bridge by heme or ferrous iron, resulting in the generation of free radicals . These radicals damage susceptible proteins and macromolecules within parasites, leading to their death . Artemisinin also regulates key factors such as apoptosis-related proteins (BAX, FASL, caspase-3), multi-drug resistance genes, cytokines (CD4+, CD8+), inflammation-related molecules (NF-κB, COX2), telomerase, and oxidative stress molecules . Additionally, artemisinin can inhibit or activate enzymes, alter gene expression, and disrupt cellular signaling pathways .
Temporal Effects in Laboratory Settings
In laboratory settings, the effects of artemisinin can change over time. Artemisinin and its derivatives have demonstrated stability and efficacy in various in vitro and in vivo studies . The long-term effects on cellular function and potential degradation of the compound are still being investigated. Studies have shown that artemisinin can maintain its antimalarial activity over extended periods, but its stability and degradation in different environments need further exploration .
Dosage Effects in Animal Models
The effects of artemisinin vary with different dosages in animal models. At therapeutic doses, artemisinin has shown efficacy in treating malaria and other diseases without significant adverse effects . At high doses, artemisinin can induce toxicity and adverse effects, including fetal death and malformations in rodents during early embryogenesis . The therapeutic window and threshold effects of artemisinin need to be carefully considered in clinical applications .
Metabolic Pathways
Artemisinin is involved in several metabolic pathways, including the mevalonate pathway and the methylerythritol phosphate pathway . These pathways produce isopentenyl diphosphate and dimethylallyl diphosphate, which are converted into farnesyl pyrophosphate by farnesyl pyrophosphate synthase . Farnesyl pyrophosphate is a key intermediate in the biosynthesis of artemisinin . Artemisinin also interacts with various enzymes and cofactors, affecting metabolic flux and metabolite levels .
Transport and Distribution
Artemisinin is transported and distributed within cells and tissues through various mechanisms. It interacts with transporters and binding proteins that facilitate its localization and accumulation . Artemisinin and its derivatives exhibit variations in distribution based on species and developmental stages . The compound’s transport and distribution are influenced by factors such as leaf age, morphology, and trichome distribution .
Subcellular Localization
Artemisinin’s subcellular localization plays a crucial role in its activity and function. Studies have shown that artemisinin accumulates in the endoplasmic reticulum and vesicles involved in intracellular trafficking . It also localizes near the mitochondria upon treatment with dihydroartemisinin . The subcellular localization of artemisinin is influenced by targeting signals and post-translational modifications that direct it to specific compartments or organelles .
Méthodes De Préparation
Voies synthétiques et conditions de réaction
L'artémisinine peut être synthétisée par plusieurs méthodes, notamment l'extraction d'Artemisia annua et l'utilisation de levures génétiquement modifiées. Le processus d'extraction consiste à isoler le composé des feuilles séchées ou des grappes de fleurs de la plante . La voie synthétique utilisant des levures génétiquement modifiées implique la biosynthèse de l'acide artémisinique, qui est ensuite converti chimiquement en artémisinine .
Méthodes de production industrielle
La production industrielle d'artémisinine implique généralement la culture d'Artemisia annua, suivie de processus d'extraction et de purification. L'extraction est souvent réalisée à l'aide de solvants tels que l'hexane ou l'éther de pétrole, et la purification est obtenue par recristallisation . Les progrès de la biotechnologie ont également permis la production d'artémisinine par fermentation microbienne, ce qui offre une approche plus durable et évolutive .
Analyse Des Réactions Chimiques
Types de réactions
L'artémisinine subit diverses réactions chimiques, notamment l'oxydation, la réduction et la substitution. La réaction la plus notable est la scission de son pont endoperoxyde, qui est cruciale pour son activité antipaludique .
Réactifs et conditions courants
Oxydation : L'artémisinine peut être oxydée à l'aide de réactifs tels que le peroxyde d'hydrogène ou l'acide m-chloroperbenzoïque.
Réduction : La réduction de l'artémisinine peut être réalisée à l'aide de borohydrure de sodium ou d'hydrure de lithium et d'aluminium.
Substitution : Les réactions de substitution impliquent souvent des nucléophiles tels que des amines ou des thiols.
Principaux produits
Les principaux produits formés à partir de ces réactions comprennent la dihydroartémisinine, l'artémether et l'artésunate. Ces dérivés conservent les propriétés antipaludiques de l'artémisinine et sont utilisés dans des thérapies combinées .
Applications de la recherche scientifique
L'artémisinine et ses dérivés ont un large éventail d'applications de recherche scientifique :
Médecine : Principalement utilisé dans le traitement du paludisme, l'artémisinine est également étudiée pour son potentiel dans le traitement d'autres infections parasitaires, du cancer et des maladies auto-immunes
Mécanisme d'action
Le mécanisme d'action de l'artémisinine implique la scission de son pont endoperoxyde par les ions fer présents dans les cellules du parasite. Cette réaction génère des espèces réactives de l'oxygène qui endommagent les protéines et les membranes vitales, entraînant la mort du parasite . L'artémisinine cible le stade schizonte du cycle de vie du parasite, ce qui est crucial pour ses effets antipaludiques rapides .
Comparaison Avec Des Composés Similaires
Composés similaires
- Dihydroartémisinine
- Artèmether
- Artésunate
- Artéether
Unicité
L'artémisinine est unique en raison de son pont endoperoxyde, qui est essentiel à son activité antipaludique. Cette caractéristique structurelle n'est pas souvent retrouvée dans d'autres composés naturels, ce qui rend l'artémisinine et ses dérivés particulièrement efficaces contre le paludisme . De plus, l'artémisinine a un spectre d'activité plus large et une élimination plus rapide des parasites par rapport à d'autres médicaments antipaludiques .
Propriétés
{ "Design of the Synthesis Pathway": "The synthesis pathway for Artemisinin involves a series of chemical reactions starting from commercially available starting materials. The key steps involve the conversion of artemisinic acid into dihydroartemisinin, which is then oxidized to form artemisinin. This pathway is efficient and widely used in industry for the large-scale production of artemisinin.", "Starting Materials": [ "Farnesyl pyrophosphate", "Methyl acrylate", "Sodium borohydride", "Hydrochloric acid", "Sodium hydroxide", "Hydrogen peroxide", "Acetic acid", "Sodium bicarbonate", "Sodium chloride", "Water" ], "Reaction": [ "1. Farnesyl pyrophosphate is reacted with methyl acrylate in the presence of sodium borohydride to form dihydroartemisinic acid.", "2. Dihydroartemisinic acid is treated with hydrochloric acid to form artemisinic acid.", "3. Artemisinic acid is treated with sodium hydroxide to form sodium artemisininate.", "4. Sodium artemisininate is oxidized with hydrogen peroxide in the presence of acetic acid to form artemisinin.", "5. The artemisinin is purified by recrystallization using a mixture of water, acetic acid, and sodium bicarbonate.", "6. The purified artemisinin is dried and packaged for use in pharmaceuticals." ] } | |
63968-64-9 | |
Formule moléculaire |
C15H22O5 |
Poids moléculaire |
282.33 g/mol |
Nom IUPAC |
(1R,13R)-1,5,9-trimethyl-11,14,15,16-tetraoxatetracyclo[10.3.1.04,13.08,13]hexadecan-10-one |
InChI |
InChI=1S/C15H22O5/c1-8-4-5-11-9(2)12(16)17-13-15(11)10(8)6-7-14(3,18-13)19-20-15/h8-11,13H,4-7H2,1-3H3/t8?,9?,10?,11?,13?,14-,15-/m1/s1 |
Clé InChI |
BLUAFEHZUWYNDE-WJRFXBFBSA-N |
SMILES isomérique |
CC1CCC2C(C(=O)OC3[C@@]24C1CC[C@](O3)(OO4)C)C |
SMILES |
CC1CCC2C(C(=O)OC3C24C1CCC(O3)(OO4)C)C |
SMILES canonique |
CC1CCC2C(C(=O)OC3C24C1CCC(O3)(OO4)C)C |
Apparence |
Solid powder |
63968-64-9 | |
Pictogrammes |
Flammable; Environmental Hazard |
Pureté |
>98% (or refer to the Certificate of Analysis) |
Durée de conservation |
>2 years if stored properly |
Solubilité |
Soluble in DMSO, not in water |
Stockage |
Dry, dark and at 0 - 4 C for short term (days to weeks) or -20 C for long term (months to years). |
Synonymes |
arteannuin artemisinin artemisinine qinghaosu quing hau sau quinghaosu |
Origine du produit |
United States |
Retrosynthesis Analysis
AI-Powered Synthesis Planning: Our tool employs the Template_relevance Pistachio, Template_relevance Bkms_metabolic, Template_relevance Pistachio_ringbreaker, Template_relevance Reaxys, Template_relevance Reaxys_biocatalysis model, leveraging a vast database of chemical reactions to predict feasible synthetic routes.
One-Step Synthesis Focus: Specifically designed for one-step synthesis, it provides concise and direct routes for your target compounds, streamlining the synthesis process.
Accurate Predictions: Utilizing the extensive PISTACHIO, BKMS_METABOLIC, PISTACHIO_RINGBREAKER, REAXYS, REAXYS_BIOCATALYSIS database, our tool offers high-accuracy predictions, reflecting the latest in chemical research and data.
Strategy Settings
Precursor scoring | Relevance Heuristic |
---|---|
Min. plausibility | 0.01 |
Model | Template_relevance |
Template Set | Pistachio/Bkms_metabolic/Pistachio_ringbreaker/Reaxys/Reaxys_biocatalysis |
Top-N result to add to graph | 6 |
Feasible Synthetic Routes
Avertissement et informations sur les produits de recherche in vitro
Veuillez noter que tous les articles et informations sur les produits présentés sur BenchChem sont destinés uniquement à des fins informatives. Les produits disponibles à l'achat sur BenchChem sont spécifiquement conçus pour des études in vitro, qui sont réalisées en dehors des organismes vivants. Les études in vitro, dérivées du terme latin "in verre", impliquent des expériences réalisées dans des environnements de laboratoire contrôlés à l'aide de cellules ou de tissus. Il est important de noter que ces produits ne sont pas classés comme médicaments et n'ont pas reçu l'approbation de la FDA pour la prévention, le traitement ou la guérison de toute condition médicale, affection ou maladie. Nous devons souligner que toute forme d'introduction corporelle de ces produits chez les humains ou les animaux est strictement interdite par la loi. Il est essentiel de respecter ces directives pour assurer la conformité aux normes légales et éthiques en matière de recherche et d'expérimentation.