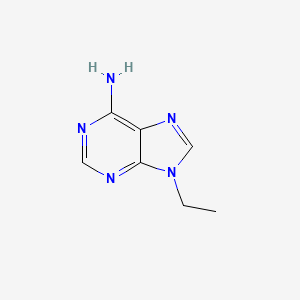
9-Éthyladénine
Vue d'ensemble
Description
La 9-Éthyl-9H-adénine est un dérivé de l'adénine, une base purique qui est un composant fondamental des acides nucléiques. Ce composé est caractérisé par la présence d'un groupe éthyle à la neuvième position de la structure de l'adénine.
Applications De Recherche Scientifique
La 9-Éthyl-9H-adénine a été largement étudiée pour son rôle d'antagoniste des récepteurs de l'adénosine. Cela en fait un outil précieux en recherche pharmacologique, en particulier dans l'étude des récepteurs de l'adénosine, qui sont impliqués dans de nombreux processus physiologiques tels que la fonction cardiaque, la neurotransmission et la réponse immunitaire . De plus, les dérivés de la 9-Éthyl-9H-adénine ont montré un potentiel dans le traitement de maladies neurologiques telles que la maladie de Parkinson .
Dans le domaine de la chimie médicinale, la 9-Éthyl-9H-adénine et ses dérivés sont explorés pour leurs activités antitumorales. Des études ont démontré leur efficacité dans l'inhibition de la prolifération de diverses lignées de cellules cancéreuses, ce qui en fait des candidats prometteurs pour le développement de médicaments anticancéreux .
Mécanisme d'action
Le principal mécanisme d'action de la 9-Éthyl-9H-adénine implique son interaction avec les récepteurs de l'adénosine. En se liant à ces récepteurs, elle agit comme un antagoniste, inhibant les effets physiologiques médiés par l'adénosine. Cela comprend la modulation de la libération de neurotransmetteurs, la régulation de la consommation d'oxygène myocardique et l'influence sur l'activité des cellules immunitaires . La capacité du composé à cibler sélectivement différents sous-types de récepteurs de l'adénosine (A1, A2A, A2B et A3) sous-tend son potentiel thérapeutique .
Mécanisme D'action
Target of Action
9-Ethyladenine, also known as 9-ethyl-9h-purin-6-amine, primarily targets the enzyme Adenine Phosphoribosyltransferase (APRT) . APRT plays a crucial role in the purine salvage pathway, which is responsible for the synthesis of adenine nucleotides from free adenine .
Mode of Action
9-Ethyladenine acts as a partial inhibitor of APRT . It interacts with the enzyme, reducing its activity and thereby affecting the production of adenine nucleotides .
Biochemical Pathways
The primary biochemical pathway affected by 9-Ethyladenine is the purine salvage pathway . By inhibiting APRT, 9-Ethyladenine disrupts the conversion of free adenine into adenine nucleotides, which are essential components of DNA, RNA, and ATP
Result of Action
The molecular and cellular effects of 9-Ethyladenine’s action are largely dependent on its inhibitory effect on APRT. By disrupting the purine salvage pathway, 9-Ethyladenine could potentially affect the synthesis of DNA, RNA, and ATP, thereby influencing a wide range of cellular processes . .
Analyse Biochimique
Biochemical Properties
9-Ethyladenine has been observed to interact with several carboxylic acids . Density functional theory (DFT) calculations combined with the quantum theory of “Atoms-in-Molecules” QTAIM have been performed to analyze the different binding modes of adenine and the energetic features of the complexes .
Cellular Effects
It has been suggested that 9-Ethyladenine derivatives bearing alkynyl chains in 2- or 8-position led to adenosine receptor antagonists .
Molecular Mechanism
It has been suggested that the interaction energies of several adenine dimers and trimers, and also the hydrogen-bonds with carboxylic acids interacting with Watson-Crick and Hoogsteen faces of adenine, have been computed and compared .
Méthodes De Préparation
La synthèse de la 9-Éthyl-9H-adénine implique généralement l'alkylation de l'adénine. Une méthode courante comprend la réaction de l'adénine avec l'iodure d'éthyle en présence d'une base telle que l'hydrure de sodium dans un solvant tel que le diméthylformamide (DMF). La réaction est effectuée à température ambiante pendant plusieurs heures pour donner de la 9-Éthyl-9H-adénine . Les méthodes de production industrielle peuvent impliquer des voies de synthèse similaires mais optimisées pour la production à grande échelle, garantissant des rendements et une pureté plus élevés.
Analyse Des Réactions Chimiques
La 9-Éthyl-9H-adénine subit diverses réactions chimiques, notamment :
Substitution nucléophile : Le composé peut participer à des réactions de substitution nucléophile, en particulier au niveau des atomes d'azote du cycle purique.
Oxydation et réduction : Bien que les réactions d'oxydation et de réduction spécifiques de la 9-Éthyl-9H-adénine soient moins documentées, sa similitude structurelle avec l'adénine suggère qu'elle peut subir des réactions redox similaires dans des conditions appropriées.
Réactions d'addition : Le composé peut également participer à des réactions d'addition avec des électrophiles, en particulier au niveau des atomes d'azote.
Les principaux produits formés à partir de ces réactions dépendent des réactifs et des conditions spécifiques utilisés. Par exemple, la substitution nucléophile avec des halogénoalcanes peut donner divers dérivés alkylés de la 9-Éthyl-9H-adénine.
Comparaison Avec Des Composés Similaires
La 9-Éthyl-9H-adénine est souvent comparée à d'autres dérivés de l'adénine et antagonistes des récepteurs de l'adénosine. Des composés similaires comprennent :
9-Éthyladénines 8-substituées : Ces dérivés présentent des modifications à la huitième position et présentent des profils de sélectivité distincts pour différents sous-types de récepteurs de l'adénosine.
9-Éthyladénines 2-substituées : Ces composés présentent des substitutions à la deuxième position et présentent des affinités variables pour les récepteurs de l'adénosine.
La singularité de la 9-Éthyl-9H-adénine réside dans sa substitution éthylique spécifique à la neuvième position, qui confère des propriétés pharmacologiques distinctes et une sélectivité des récepteurs par rapport à d'autres dérivés de l'adénine.
Conclusion
La 9-Éthyl-9H-adénine est un composé d'un intérêt considérable en recherche scientifique en raison de sa structure chimique unique et de ses propriétés pharmacologiques. Sa synthèse, sa réactivité et ses applications en chimie médicinale mettent en évidence son potentiel en tant qu'outil précieux dans le développement de médicaments et les interventions thérapeutiques.
Propriétés
IUPAC Name |
9-ethylpurin-6-amine | |
---|---|---|
Source | PubChem | |
URL | https://pubchem.ncbi.nlm.nih.gov | |
Description | Data deposited in or computed by PubChem | |
InChI |
InChI=1S/C7H9N5/c1-2-12-4-11-5-6(8)9-3-10-7(5)12/h3-4H,2H2,1H3,(H2,8,9,10) | |
Source | PubChem | |
URL | https://pubchem.ncbi.nlm.nih.gov | |
Description | Data deposited in or computed by PubChem | |
InChI Key |
MUIPLRMGAXZWSQ-UHFFFAOYSA-N | |
Source | PubChem | |
URL | https://pubchem.ncbi.nlm.nih.gov | |
Description | Data deposited in or computed by PubChem | |
Canonical SMILES |
CCN1C=NC2=C(N=CN=C21)N | |
Source | PubChem | |
URL | https://pubchem.ncbi.nlm.nih.gov | |
Description | Data deposited in or computed by PubChem | |
Molecular Formula |
C7H9N5 | |
Source | PubChem | |
URL | https://pubchem.ncbi.nlm.nih.gov | |
Description | Data deposited in or computed by PubChem | |
DSSTOX Substance ID |
DTXSID50181616 | |
Record name | 9-Ethyladenine | |
Source | EPA DSSTox | |
URL | https://comptox.epa.gov/dashboard/DTXSID50181616 | |
Description | DSSTox provides a high quality public chemistry resource for supporting improved predictive toxicology. | |
Molecular Weight |
163.18 g/mol | |
Source | PubChem | |
URL | https://pubchem.ncbi.nlm.nih.gov | |
Description | Data deposited in or computed by PubChem | |
CAS No. |
2715-68-6 | |
Record name | 9-Ethyladenine | |
Source | ChemIDplus | |
URL | https://pubchem.ncbi.nlm.nih.gov/substance/?source=chemidplus&sourceid=0002715686 | |
Description | ChemIDplus is a free, web search system that provides access to the structure and nomenclature authority files used for the identification of chemical substances cited in National Library of Medicine (NLM) databases, including the TOXNET system. | |
Record name | 9-Ethyladenine | |
Source | DTP/NCI | |
URL | https://dtp.cancer.gov/dtpstandard/servlet/dwindex?searchtype=NSC&outputformat=html&searchlist=14580 | |
Description | The NCI Development Therapeutics Program (DTP) provides services and resources to the academic and private-sector research communities worldwide to facilitate the discovery and development of new cancer therapeutic agents. | |
Explanation | Unless otherwise indicated, all text within NCI products is free of copyright and may be reused without our permission. Credit the National Cancer Institute as the source. | |
Record name | 9-Ethyladenine | |
Source | EPA DSSTox | |
URL | https://comptox.epa.gov/dashboard/DTXSID50181616 | |
Description | DSSTox provides a high quality public chemistry resource for supporting improved predictive toxicology. | |
Synthesis routes and methods
Procedure details
Retrosynthesis Analysis
AI-Powered Synthesis Planning: Our tool employs the Template_relevance Pistachio, Template_relevance Bkms_metabolic, Template_relevance Pistachio_ringbreaker, Template_relevance Reaxys, Template_relevance Reaxys_biocatalysis model, leveraging a vast database of chemical reactions to predict feasible synthetic routes.
One-Step Synthesis Focus: Specifically designed for one-step synthesis, it provides concise and direct routes for your target compounds, streamlining the synthesis process.
Accurate Predictions: Utilizing the extensive PISTACHIO, BKMS_METABOLIC, PISTACHIO_RINGBREAKER, REAXYS, REAXYS_BIOCATALYSIS database, our tool offers high-accuracy predictions, reflecting the latest in chemical research and data.
Strategy Settings
Precursor scoring | Relevance Heuristic |
---|---|
Min. plausibility | 0.01 |
Model | Template_relevance |
Template Set | Pistachio/Bkms_metabolic/Pistachio_ringbreaker/Reaxys/Reaxys_biocatalysis |
Top-N result to add to graph | 6 |
Feasible Synthetic Routes
ANone: While 9-ethyladenine can bind to adenosine receptors, it primarily acts as an antagonist rather than an agonist. [] This means it binds to the receptors but does not activate them in the same way as adenosine itself. Specific derivatives of 9-ethyladenine have shown varying affinities for different adenosine receptor subtypes (A1, A2A, A2B, A3). [, , , ]
ANone: Yes, the ethyl group at the N9 position of adenine influences its ability to form hydrogen bonds. For instance, it can hinder the formation of certain hydrogen-bonded dimers with uracil derivatives compared to unsubstituted adenine. [, , ]
ANone: Studies show that 9-ethyladenine forms stable complexes with riboflavin tetraacetate and tetrabutyrate in chloroform. [] These complexes involve cyclic hydrogen bonding between the adenine amino group and the isoalloxazine ring of riboflavin. Barbiturates can disrupt this complex formation, highlighting potential interactions relevant to biological systems. []
ANone: The molecular formula of 9-ethyladenine is C7H9N5. It has a molecular weight of 163.18 g/mol.
ANone: Several spectroscopic methods are valuable for studying 9-ethyladenine. These include:
- NMR Spectroscopy: Provides information about structure, self-association, and complex formation with other molecules. [, , , , , , ]
- Infrared Spectroscopy: Useful for identifying hydrogen bonding patterns and characterizing interactions with other molecules. [, , , , , , , , ]
- UV-Vis Spectroscopy: Used to study complex formation and the influence of solvent environment on 9-ethyladenine derivatives. [, , , ]
- Mass Spectrometry: Can be used to analyze 9-ethyladenine and its metabolites in biological samples. [, ]
ANone: The self-association of 9-ethyladenine is influenced by the solvent. For example, it forms hydrogen-bonded dimers in concentrated chloroform solutions but shows minimal self-association in dilute solutions. [] This behavior is also observed in other nonpolar solvents like cyclohexane. []
ANone: 9-Ethyladenine is often used as a template molecule in molecular imprinting studies for creating synthetic receptors with selectivity for adenine and related compounds. [, ] This process involves forming a polymer network around the template molecule, creating cavities that can subsequently rebind to the target molecule or its analogs.
ANone: Computational chemistry, including density functional theory (DFT) calculations, has been employed to:
- Predict acidity: Calculate the gas-phase acidities of 9-ethyladenine and other adenine derivatives, aiding in understanding their reactivity. [, ]
- Analyze spectroscopic data: Interpret infrared and UV-Vis spectra, allowing for structural assignments of different dimer structures formed by 9-ethyladenine in solution. [, ]
- Study molecular interactions: Investigate hydrogen bonding patterns and base-pairing preferences in complexes with other molecules. []
ANone: Research indicates that substituents on the adenine ring significantly influence the type of adenine-uracil dimers formed. For instance:
- 2-Chloro-9-ethyladenine and N2-(dimethylamino)-9-ethyladenine predominantly form Hoogsteen-type dimers. []
- 8-Bromo-9-ethyladenine, N2-(methylamino)-9-ethyladenine, and 2-amino-9-ethylpurine favor Watson-Crick-type dimers. []
ANone: Studies indicate that 8-bromo-9-ethyladenine exhibits enhanced binding affinity for the A2A adenosine receptor compared to unsubstituted 9-ethyladenine. This highlights the potential for specific structural modifications to modulate the biological activity of adenine derivatives. [, ]
Avertissement et informations sur les produits de recherche in vitro
Veuillez noter que tous les articles et informations sur les produits présentés sur BenchChem sont destinés uniquement à des fins informatives. Les produits disponibles à l'achat sur BenchChem sont spécifiquement conçus pour des études in vitro, qui sont réalisées en dehors des organismes vivants. Les études in vitro, dérivées du terme latin "in verre", impliquent des expériences réalisées dans des environnements de laboratoire contrôlés à l'aide de cellules ou de tissus. Il est important de noter que ces produits ne sont pas classés comme médicaments et n'ont pas reçu l'approbation de la FDA pour la prévention, le traitement ou la guérison de toute condition médicale, affection ou maladie. Nous devons souligner que toute forme d'introduction corporelle de ces produits chez les humains ou les animaux est strictement interdite par la loi. Il est essentiel de respecter ces directives pour assurer la conformité aux normes légales et éthiques en matière de recherche et d'expérimentation.