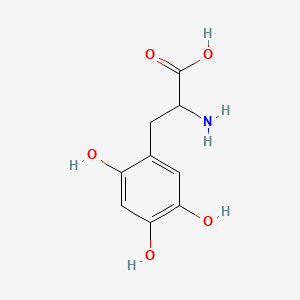
6-Hydroxy-DL-DOPA
Vue d'ensemble
Description
6-Hydroxy-DOPA, également connu sous le nom de 2,4,5-trihydroxyphénylalanine, est une neurotoxine catécholamine et une excitotoxine endogène. Il s'agit d'un dérivé de l'acide aminé tyrosine et il est structurellement similaire à la dopamine. Ce composé est principalement connu pour ses effets neurotoxiques sélectifs sur les neurones noradrénergiques, ce qui en fait un outil précieux dans la recherche neurobiologique .
Applications De Recherche Scientifique
6-Hydroxy-DOPA has a wide range of scientific research applications, including:
Neurobiological Research: It is used to study the selective destruction of noradrenergic neurons, providing insights into neurodegenerative diseases such as Parkinson’s disease
Cancer Research: 6-Hydroxy-DOPA acts as an allosteric inhibitor of RAD52 and APE1, making it useful in studying the proliferation of BRCA-deficient cancer cells
DNA Repair Studies: It inhibits RAD52 binding to single-strand DNA binding domains, contributing to research on DNA repair mechanisms
Pharmacological Studies: The compound is used to investigate the effects of neurotoxins and excitotoxins on neuronal function and survival
Mécanisme D'action
Target of Action
6-Hydroxy-DL-DOPA, also known as 6-Hydroxydopa or 2,4,5-Trihydroxyphenylalanine, primarily targets two proteins: RAD52 and APE1 .
- RAD52 : This protein plays a crucial role in DNA repair, particularly in the homologous recombination pathway .
- APE1 : Also known as apurinic/apyrimidinic endonuclease, APE1 is a nuclease that is essential for the DNA base excision repair pathway .
Mode of Action
This compound acts as an allosteric inhibitor of RAD52, inhibiting RAD52’s binding to single-strand DNA binding domains . It also inhibits the repair-function activity of APE1 .
Biochemical Pathways
The primary biochemical pathways affected by this compound are the DNA repair pathways . By inhibiting RAD52 and APE1, this compound disrupts the homologous recombination pathway and the DNA base excision repair pathway, respectively .
Pharmacokinetics
It is soluble in 1m hcl, suggesting that it may be absorbed in the acidic environment of the stomach .
Result of Action
The inhibition of RAD52 and APE1 by this compound leads to a disruption in DNA repair, which can result in DNA damage and genomic instability . This compound selectively inhibits the proliferation of BRCA-deficient cancer cells in vitro .
Action Environment
It is known that the compound should be stored at -20°c and that solutions should be freshly prepared and protected from exposure to light .
Analyse Biochimique
Biochemical Properties
6-Hydroxy-DL-DOPA plays a crucial role in biochemical reactions, particularly as an inhibitor of certain enzymes. It is known to inhibit RAD52, a protein involved in DNA repair, by binding to its single-strand DNA binding domains . Additionally, this compound inhibits APE1 (apurinic/apyrimidinic endonuclease), which is essential for the DNA base excision repair pathway . These interactions highlight the compound’s potential in influencing DNA repair mechanisms and its relevance in cancer research, particularly in BRCA-deficient cancer cells .
Cellular Effects
This compound has notable effects on various cell types and cellular processes. It selectively inhibits the proliferation of BRCA-deficient cancer cells by disrupting the RAD52 protein’s ability to bind to single-strand DNA . This disruption leads to impaired DNA repair and increased cell death in these cancer cells. Furthermore, this compound has been shown to affect noradrenergic neurons, leading to neurotoxicity and the destruction of noradrenergic nerve terminals in peripheral organs and tissues . This neurotoxic effect is particularly significant in studies related to neurodegenerative diseases.
Molecular Mechanism
The molecular mechanism of this compound involves its interaction with specific biomolecules and enzymes. As an allosteric inhibitor of RAD52, this compound binds to the protein and transforms its oligomeric ring structures into dimers, thereby abolishing the ssDNA binding channel . This inhibition prevents RAD52 from participating in DNA repair processes, leading to increased sensitivity of BRCA-deficient cells to DNA damage. Additionally, this compound inhibits APE1, further compromising the DNA repair pathways and enhancing its cytotoxic effects on cancer cells .
Temporal Effects in Laboratory Settings
In laboratory settings, the effects of this compound can change over time. The compound is relatively stable when stored at -20°C and protected from light . Its neurotoxic effects on noradrenergic neurons are not permanent, as the sympathetic nervous system can regenerate and reinnervate the affected areas within approximately six weeks . This temporal aspect is crucial for understanding the long-term implications of this compound in both in vitro and in vivo studies.
Dosage Effects in Animal Models
The effects of this compound vary with different dosages in animal models. At high doses, the compound can produce near-total noradrenergic denervation of the hippocampus, neocortex, and cerebellum in rodents . This extensive denervation leads to significant neurotoxicity and can be lethal at extremely high doses. Conversely, lower doses may result in partial denervation and allow for the study of regenerative processes in the nervous system.
Metabolic Pathways
This compound is involved in several metabolic pathways, particularly those related to catecholamine metabolism. It is a precursor to the neurotoxin 6-hydroxydopamine, which is formed by the action of L-aromatic amino acid decarboxylase . This conversion is significant in studies of neurodegenerative diseases, as 6-hydroxydopamine is used to model Parkinson’s disease in animal studies. Additionally, this compound can be further oxidized to form quinones, which contribute to its neurotoxic effects .
Transport and Distribution
Within cells and tissues, this compound is transported and distributed through various mechanisms. It interacts with specific transporters and binding proteins that facilitate its movement across cellular membranes . The compound’s distribution is influenced by its solubility and stability, with higher concentrations observed in regions with active catecholamine metabolism. This distribution pattern is essential for understanding its localized effects on cellular function.
Subcellular Localization
The subcellular localization of this compound plays a critical role in its activity and function. The compound is primarily localized in the cytoplasm, where it interacts with enzymes and proteins involved in DNA repair and catecholamine metabolism . Its localization is influenced by targeting signals and post-translational modifications that direct it to specific cellular compartments. Understanding this localization is crucial for elucidating the precise mechanisms of its biochemical and cellular effects.
Méthodes De Préparation
Voies de Synthèse et Conditions de Réaction : Le 6-Hydroxy-DOPA peut être synthétisé par différentes voies chimiques. Une méthode courante implique l'hydroxylation de la L-DOPA (3,4-dihydroxyphénylalanine) en utilisant des agents hydroxylants spécifiques dans des conditions contrôlées. La réaction nécessite généralement un catalyseur et est effectuée en milieu aqueux afin de garantir la stabilité du produit hydroxylé .
Méthodes de Production Industrielle : La production industrielle du 6-Hydroxy-DOPA implique une synthèse à grande échelle utilisant des conditions de réaction optimisées pour maximiser le rendement et la pureté. Le processus comprend l'utilisation de réactifs de haute pureté et de techniques de purification avancées pour isoler le produit souhaité. Des mesures de contrôle qualité sont mises en œuvre pour garantir la cohérence et la sécurité du produit final .
Analyse Des Réactions Chimiques
Types de Réactions : Le 6-Hydroxy-DOPA subit plusieurs types de réactions chimiques, notamment :
Oxydation : Il s'oxyde facilement pour former des espèces réactives de l'oxygène (ROS) telles que le superoxyde et le peroxyde d'hydrogène. .
Substitution : Des réactions de substitution peuvent se produire au niveau des groupes hydroxyle, conduisant à la formation de divers dérivés substitués.
Réactifs et Conditions Communs :
Oxydation : Les agents oxydants courants comprennent l'oxygène moléculaire, le peroxyde d'hydrogène et les catalyseurs métalliques tels que les ions fer ou cuivre
Principaux Produits Formés :
Produits d'Oxydation : Quinones, dopachrome et dopaminechrome
Produits de Réduction : Dérivés de catécholamine réduits.
Produits de Substitution : Diverses catécholamines substituées.
4. Applications de Recherche Scientifique
Le 6-Hydroxy-DOPA a un large éventail d'applications de recherche scientifique, notamment :
Recherche Neurobiologique : Il est utilisé pour étudier la destruction sélective des neurones noradrénergiques, fournissant des informations sur les maladies neurodégénératives telles que la maladie de Parkinson
Recherche sur le Cancer : Le 6-Hydroxy-DOPA agit comme un inhibiteur allostérique de RAD52 et APE1, ce qui le rend utile dans l'étude de la prolifération des cellules cancéreuses déficientes en BRCA
Études de Réparation de l'ADN : Il inhibe la liaison de RAD52 aux domaines de liaison de l'ADN monobrin, contribuant à la recherche sur les mécanismes de réparation de l'ADN
Études Pharmacologiques : Le composé est utilisé pour étudier les effets des neurotoxines et des excitotoxines sur la fonction et la survie neuronale
5. Mécanisme d'Action
Le 6-Hydroxy-DOPA exerce ses effets par plusieurs mécanismes :
Neurotoxicité : Il cible sélectivement les neurones noradrénergiques en générant des espèces réactives de l'oxygène (ROS) et des quinones toxiques, conduisant à un stress oxydatif et à des dommages neuronaux
Inhibition de la Réparation de l'ADN : Il inhibe l'activité de RAD52 et APE1, protéines cruciales impliquées dans les voies de réparation de l'ADN
Excitotoxicité : Le composé agit comme une excitotoxine au niveau des récepteurs non-NMDA, provoquant une excitation neuronale excessive et une mort cellulaire subséquente.
Comparaison Avec Des Composés Similaires
Le 6-Hydroxy-DOPA est souvent comparé à d'autres dérivés de catécholamine, tels que :
6-Hydroxydopamine (6-OHDA) : Les deux composés sont des neurotoxines, mais le 6-Hydroxy-DOPA est plus sélectif pour les neurones noradrénergiques
Dopamine : Contrairement à la dopamine, le 6-Hydroxy-DOPA possède des propriétés neurotoxiques et est principalement utilisé en milieu de recherche
L-DOPA : Alors que la L-DOPA est un précurseur de la dopamine et est utilisée dans le traitement de la maladie de Parkinson, le 6-Hydroxy-DOPA est utilisé pour modéliser les conditions neurodégénératives
Propriétés
IUPAC Name |
2-amino-3-(2,4,5-trihydroxyphenyl)propanoic acid | |
---|---|---|
Source | PubChem | |
URL | https://pubchem.ncbi.nlm.nih.gov | |
Description | Data deposited in or computed by PubChem | |
InChI |
InChI=1S/C9H11NO5/c10-5(9(14)15)1-4-2-7(12)8(13)3-6(4)11/h2-3,5,11-13H,1,10H2,(H,14,15) | |
Source | PubChem | |
URL | https://pubchem.ncbi.nlm.nih.gov | |
Description | Data deposited in or computed by PubChem | |
InChI Key |
YLKRUSPZOTYMAT-UHFFFAOYSA-N | |
Source | PubChem | |
URL | https://pubchem.ncbi.nlm.nih.gov | |
Description | Data deposited in or computed by PubChem | |
Canonical SMILES |
C1=C(C(=CC(=C1O)O)O)CC(C(=O)O)N | |
Source | PubChem | |
URL | https://pubchem.ncbi.nlm.nih.gov | |
Description | Data deposited in or computed by PubChem | |
Molecular Formula |
C9H11NO5 | |
Source | PubChem | |
URL | https://pubchem.ncbi.nlm.nih.gov | |
Description | Data deposited in or computed by PubChem | |
DSSTOX Substance ID |
DTXSID00943928 | |
Record name | 2,5-Dihydroxytyrosine | |
Source | EPA DSSTox | |
URL | https://comptox.epa.gov/dashboard/DTXSID00943928 | |
Description | DSSTox provides a high quality public chemistry resource for supporting improved predictive toxicology. | |
Molecular Weight |
213.19 g/mol | |
Source | PubChem | |
URL | https://pubchem.ncbi.nlm.nih.gov | |
Description | Data deposited in or computed by PubChem | |
CAS No. |
21373-30-8 | |
Record name | 3-(2,4,5-Trihydroxyphenyl)-DL-alanine | |
Source | CAS Common Chemistry | |
URL | https://commonchemistry.cas.org/detail?cas_rn=21373-30-8 | |
Description | CAS Common Chemistry is an open community resource for accessing chemical information. Nearly 500,000 chemical substances from CAS REGISTRY cover areas of community interest, including common and frequently regulated chemicals, and those relevant to high school and undergraduate chemistry classes. This chemical information, curated by our expert scientists, is provided in alignment with our mission as a division of the American Chemical Society. | |
Explanation | The data from CAS Common Chemistry is provided under a CC-BY-NC 4.0 license, unless otherwise stated. | |
Record name | 6-Hydroxydopa | |
Source | ChemIDplus | |
URL | https://pubchem.ncbi.nlm.nih.gov/substance/?source=chemidplus&sourceid=0021373308 | |
Description | ChemIDplus is a free, web search system that provides access to the structure and nomenclature authority files used for the identification of chemical substances cited in National Library of Medicine (NLM) databases, including the TOXNET system. | |
Record name | 2,5-Dihydroxytyrosine | |
Source | EPA DSSTox | |
URL | https://comptox.epa.gov/dashboard/DTXSID00943928 | |
Description | DSSTox provides a high quality public chemistry resource for supporting improved predictive toxicology. | |
Record name | 6-HYDROXYDOPA, DL- | |
Source | FDA Global Substance Registration System (GSRS) | |
URL | https://gsrs.ncats.nih.gov/ginas/app/beta/substances/9HBL9XYQ8O | |
Description | The FDA Global Substance Registration System (GSRS) enables the efficient and accurate exchange of information on what substances are in regulated products. Instead of relying on names, which vary across regulatory domains, countries, and regions, the GSRS knowledge base makes it possible for substances to be defined by standardized, scientific descriptions. | |
Explanation | Unless otherwise noted, the contents of the FDA website (www.fda.gov), both text and graphics, are not copyrighted. They are in the public domain and may be republished, reprinted and otherwise used freely by anyone without the need to obtain permission from FDA. Credit to the U.S. Food and Drug Administration as the source is appreciated but not required. | |
Retrosynthesis Analysis
AI-Powered Synthesis Planning: Our tool employs the Template_relevance Pistachio, Template_relevance Bkms_metabolic, Template_relevance Pistachio_ringbreaker, Template_relevance Reaxys, Template_relevance Reaxys_biocatalysis model, leveraging a vast database of chemical reactions to predict feasible synthetic routes.
One-Step Synthesis Focus: Specifically designed for one-step synthesis, it provides concise and direct routes for your target compounds, streamlining the synthesis process.
Accurate Predictions: Utilizing the extensive PISTACHIO, BKMS_METABOLIC, PISTACHIO_RINGBREAKER, REAXYS, REAXYS_BIOCATALYSIS database, our tool offers high-accuracy predictions, reflecting the latest in chemical research and data.
Strategy Settings
Precursor scoring | Relevance Heuristic |
---|---|
Min. plausibility | 0.01 |
Model | Template_relevance |
Template Set | Pistachio/Bkms_metabolic/Pistachio_ringbreaker/Reaxys/Reaxys_biocatalysis |
Top-N result to add to graph | 6 |
Feasible Synthetic Routes
Q1: How does 6-hydroxydopa (TOPA) exert its neurotoxic effects?
A1: TOPA's neurotoxicity is primarily attributed to two mechanisms:
- Excitotoxicity: TOPA, particularly its oxidized form, topa quinone, acts as a potent non-N-methyl-D-aspartate (non-NMDA) glutamatergic agonist. [] This leads to excessive neuronal excitation and subsequent cell death. [, ]
- Oxidative Stress: TOPA readily undergoes autoxidation, generating reactive oxygen species (ROS) such as hydrogen peroxide (H2O2), superoxide radical (O2•-), and hydroxyl radical (•OH). [, ] These ROS cause oxidative damage to cellular components like lipids, proteins, and DNA, ultimately contributing to neuronal death. [, ]
Q2: Does 6-hydroxydopamine (6-OHDA) play a role in TOPA-induced neurotoxicity?
A2: While TOPA itself is neurotoxic, its in vivo conversion to 6-OHDA might contribute to its overall toxicity. 6-OHDA is a well-known neurotoxin that causes degeneration of noradrenergic nerve terminals. [] Some studies suggest that TOPA might be converted to 6-OHDA in vivo, further exacerbating its neurotoxic effects. []
Q3: How does TOPA affect norepinephrine (NE) levels in the brain?
A3: TOPA administration leads to a complex pattern of NE alterations in different brain regions:
- Depletion: In regions supplied by the dorsal noradrenergic bundle, like the neocortex and hippocampus, TOPA causes significant and long-lasting NE depletion. [] This depletion is likely due to the degeneration of noradrenergic neurons and impaired NE uptake. []
- Elevation: Conversely, TOPA can elevate NE levels in other areas like the cerebellum. [, ] This increase is attributed to enhanced intraneuronal NE storage rather than increased nerve endings. []
Q4: What is the molecular formula and weight of 6-hydroxydopa?
A4: The molecular formula of 6-hydroxydopa is C9H11NO5, and its molecular weight is 213.19 g/mol.
Q5: What are the key spectroscopic characteristics of 6-hydroxydopa and its derivatives?
A5:
- 6-Hydroxydopa: Exhibits a UV absorbance maximum (λmax) at 291 nm. []
- Topa Quinone: Displays a UV absorbance maximum (λmax) at 460 nm in the presence of amines. []
- 6-Hydroxydopa Radical: Characterized by a sharp peak at 440 nm, shifting to 460 nm upon amine addition. []
- Phenylhydrazine and p-Nitrophenylhydrazine Derivatives: Exhibit distinct resonance Raman spectra that are consistent with the presence of topa quinone. []
Q6: What is the biological role of 6-hydroxydopa?
A6: TOPA, in its oxidized quinone form (TPQ), serves as an essential cofactor in copper amine oxidases (CAOs). [, , , ] CAOs are enzymes that catalyze the oxidative deamination of primary amines. []
Q7: How does topa quinone function as a cofactor in CAOs?
A7: Topa quinone acts as a redox-active cofactor, cycling between its oxidized (quinone) and reduced (benzenetriol) forms during the catalytic cycle of CAOs. It participates in the transfer of electrons from the amine substrate to molecular oxygen, ultimately leading to the formation of an aldehyde, ammonia, and hydrogen peroxide. [, , , ]
Q8: Can copper amine oxidases function without the topa quinone cofactor?
A8: No, the topa quinone cofactor is essential for the catalytic activity of copper amine oxidases. Studies with copper-free apoenzymes demonstrate that they cannot oxidize substrates efficiently. [, ]
Q9: How is topa quinone generated in CAOs?
A9: Topa quinone is produced through post-translational modification of a specific tyrosine residue within the CAO active site. This process requires both molecular oxygen and the enzyme-bound copper ion. [, , ]
Q10: What are the main toxic effects of 6-hydroxydopa?
A10: TOPA is a strong neurotoxin, primarily targeting catecholaminergic neurons. [, , ] Its toxicity is linked to both its ability to induce oxidative stress [, ] and its action as an excitotoxic agent, particularly in its oxidized topa quinone form. [, ]
Q11: Are there any protective strategies against TOPA-induced neurotoxicity?
A11: Research suggests that certain compounds may offer protection against TOPA-induced damage:
- Antioxidants: Compounds like norepinephrine can neutralize the cytotoxic effects of TOPA and its related compounds by scavenging free radicals generated during autoxidation. []
- NMDA Receptor Antagonists: Antagonists of non-NMDA glutamate receptors, such as 6-cyano-7-nitroquinoxaline-2,3-dione (CNQX), can block TOPA's excitotoxic effects and protect neurons from cell death. [, , ]
- Ganglioside GM1: This neuroprotective agent has demonstrated efficacy in safeguarding neurons against TOPA-induced degeneration. []
Avertissement et informations sur les produits de recherche in vitro
Veuillez noter que tous les articles et informations sur les produits présentés sur BenchChem sont destinés uniquement à des fins informatives. Les produits disponibles à l'achat sur BenchChem sont spécifiquement conçus pour des études in vitro, qui sont réalisées en dehors des organismes vivants. Les études in vitro, dérivées du terme latin "in verre", impliquent des expériences réalisées dans des environnements de laboratoire contrôlés à l'aide de cellules ou de tissus. Il est important de noter que ces produits ne sont pas classés comme médicaments et n'ont pas reçu l'approbation de la FDA pour la prévention, le traitement ou la guérison de toute condition médicale, affection ou maladie. Nous devons souligner que toute forme d'introduction corporelle de ces produits chez les humains ou les animaux est strictement interdite par la loi. Il est essentiel de respecter ces directives pour assurer la conformité aux normes légales et éthiques en matière de recherche et d'expérimentation.